Hydration and Determination of Hydrodynamic Radii of Zinc Sulphate Heptahydrate: A Viscometric and Conductometric Study
Narain Datt Kandpal1, Anchal Aneja2*
, Renu Loshali3
and Asha Kandpal1
1Physical Chemistry Laboratory, Department of Chemistry, Kumaun University, SSJ Campus, Almora, Uttarakhand, India.
2Department of Chemistry, D.S.B. Campus, Kumaun University, Nainital, Uttarakhand, India.
3Department of Chemistry, Shri Ram Singh Dhoni Rajkiya Mahavidhalaya, Jainti (Almora) Soban Singh Jeena University, Uttarakhand, India.
Corresponding Author E-mail:anchalaneja@kunainital.ac.in
DOI : http://dx.doi.org/10.13005/ojc/400606
Article Received on : 02 Oct 2024
Article Accepted on :
Article Published : 12 Dec 2024
Reviewed by: Dr. Sri Atun
Second Review by: Dr. Asif
Final Approval by: Dr. Dinesh Chand Sharma
The viscosity and conductivity data of zinc sulphate heptahydrate are measured at temperature 303K and 308K in aqueous solution over the concentration range 0.1 moldm-3 to 1.0 moldm-3. The variation in viscosity with the molarity of the salt in water depicts nature of hydration of ions taking place in three different regions low concentration region, middle concentration region and higher concentration region of solution. The value of dissociation constant (Kc), degree of dissociation (α), molar conductivity at infinite dilution ( ) and hydrodynamic radii (r) have been calculated at both the temperatures. Results indicated that hydrophilic interactions are dominant in the hydration process of zinc sulphate heptahydrate followed by the formation of hydrated ion-pairs surrounded by the water molecules.
KEYWORDS:Conductivity; Dissociation Constant; Hydrodynamic radii; Solute-Solvent interaction; Viscosity; Zinc sulphate heptahydrate
Download this article as:
Copy the following to cite this article: Kandpal N. D, Aneja A, Loshali R, Kandpal A. Hydration and Determination of Hydrodynamic Radii of Zinc Sulphate Heptahydrate: A Viscometric and Conductometric Study. Orient J Chem 2024;40(6). |
Copy the following to cite this URL: Kandpal N. D, Aneja A, Loshali R, Kandpal A. Hydration and Determination of Hydrodynamic Radii of Zinc Sulphate Heptahydrate: A Viscometric and Conductometric Study. Orient J Chem 2024;40(6). Available from: https://bit.ly/41E8Wl9 |
Introduction
Viscosity and conductivity of the solutions are of fundamental importance for understanding the hydration behaviour of dissolved electrolytes in water. Survey of literature on viscometric study showed that the hydrated salts of cobalt sulphate, nickel sulphate, copper sulphate, zinc sulphate and magnesium sulphate act as structure maker while the anhydrous salts act as structure breaker in water1. The removal of water of crystallization changes the hydration behaviour of these salts. In the hydration process, hydrophobic hydration and hydrophilic hydration of ions takes place which affect each other leads to the destructive or cooperative interactions. There is a great interest in viscometric and conductometric studies on hydration of transition metal salts due to their common involvement in chemical and biological systems2-5. Our attention have been focused on the hydration behaviour of the ions because there is a report in which the water of crystallization becomes essential in the synthetic method for nanoparticles6. There is another report in which hydration has important role in the formation of layered hydroxide acetate salts represented by M(OH)1-x(CH3COO)x.nH2O(M=Co, Ni, Co-Ni and Zn)7. In the solvation of any solute in solvent depends upon its characteristics like ionic, polar, non-polar, associative, dissociative, neutral nature of species in solution and concentration of the solute. The behavior of the solute in solution can be observed by the measurement of transport properties like viscosity, surface tension, conductance and density8-10. These properties are useful for the measurement of intermolecular interactions present in solution. The viscometric and conductometric studies are important to access the various aspects of physicochemical behaviour of solute in solvent such as molecular association, dissociation, ion-pair formation and complex formation11-13. Very recently we have made an effort to investigate the hydration behavior of copper sulphate pentahydrate by measuring the viscosity and conductance of solution having different concentration at temperatures 308K and 313K14. It was found in the study that the formation of hydrated ion-pair takes place in the process of hydration of the salt. Like copper, zinc also belongs to the class of transition metals having atomic no. 30 next to the copper having atomic no. 29 and have seven molecules of crystalline water. The present study has been undertaken in order to investigate the hydration behaviour of zinc sulphate heptahydrate using viscometric and conductometric measurements under same experimental conditions of copper sulphate pentahydrate.
Materials and Methods
Chemicals and Materials
Double distilled water was used for the preparation of solutions. Zinc sulphate heptahydrate was used has grade Qualigen. The pyknometer having capacity 1×10-5m3 was used for the measurement of the density. The thermostat water bath was used to maintain the constant temperature with the variation of ±0.01K. A citizen makes electronic balance with an accuracy of ±1×10-7Kg was used for the measurement of the weight. Ubbelohode-type capillary viscometer (Agarwal scientific, Agra) was used for the viscosity measurement. The flow time of the solution was measured with the help of stop watch with a resolution of 0.01 sec. Digital conductivity meter, Systonic 306 make and conductivity cell having platinized electrodes was used for the measurement of the conductivity.
Methods
The purity of distilled water was checked by measuring the specific conductance having always in the order of 2 x 10-4 Ω-1m-1. The solutions of desired strength were prepared by dissolving the calculated weight of the sample into distilled water during the study. The pyknometer was calibrated with the distilled water having densities 0.997g/cm-3 and 0.9942 g/cm-3 at 298K and 308K respectively. At particular temperature the densities of the solutions were measured from the mass of the solution in the pyknometer after reaching thermal equilibria in the thermostat water bath capable of maintaining the constant temperature. The viscometer was calibrated with double distilled water and flow time was measured for each concentration solution at temperature 303K and 308K. The concurrent and reproducible time was taken as the final efflux time. The conductivity meter was always calibrated with the standard KCl solution. The accuracy of conductivity meter was 0.01 mΩ-1m-1 during the study, the results were taken after the checking of constant reading.
Results
The experimental results of density, specific conductance and viscosity of zinc sulphate heptahydrate solutions in water at temperature 303K and 308K are given in Table 1.
Table 1: Experimental values of density (d1), relative viscosity (ƞ/ƞo), and specific conductance (Ks) of aqueous solution of zinc sulphate heptahydrate at 303K and 308K
S. No. |
Conc(c) (moldm-3) |
Density, relative viscosity and specific conductance |
|||||
(303K) |
(308K) |
||||||
(d1) (gmL-1) |
(ƞ/ƞo) |
(Ks x 102) (mΩ-1m-1) |
(d1) (gmL-1) |
(ƞ/ƞo) |
(Ks x 102) (mΩ-1m-1) |
||
01 |
0.1 |
1.0003 |
1.0299 |
6.51 |
1.0000 |
1.0530 |
5.95 |
02 |
0.2 |
1.0008 |
1.1301 |
10.81 |
1.0005 |
1.1827 |
9.86 |
03 |
0.3 |
0.9988 |
1.2440 |
13.62 |
0.9983 |
1.2500 |
12.34 |
04 |
0.4 |
1.0369 |
1.3430 |
16.44 |
1.0364 |
1.3125 |
15.09 |
05 |
0.5 |
1.0582 |
1.4409 |
16.90 |
1.0580 |
1.4113 |
15.45 |
06 |
0.6 |
1.0778 |
1.5393 |
18.40 |
1.0774 |
1.5100 |
16.30 |
07 |
0.7 |
1.0547 |
1.5588 |
20.00 |
1.0544 |
1.5490 |
18.40 |
08 |
0.8 |
1.1105 |
1.7519 |
21.20 |
1.1101 |
1.7433 |
19.20 |
09 |
0.9 |
1.1029 |
1.9047 |
22.60 |
1.1029 |
1.8251 |
20.50 |
10 |
1.0 |
1.1302 |
2.0270 |
22.80 |
1.1298 |
1.9460 |
20.62 |
Water |
– |
0.9872 |
– |
0.006 |
0.9870 |
– |
0.0055 |
Discussion
The viscosity data collected in Table 1 were used to calculate the values of η/ηo-1/c,
η/ηo-1/√c for each concentration of zinc sulphate heptahydrate in the concentration range 0.1 moldm-3 to 1.0 moldm-3 at both the temperature 303K and 308K. The specific conductance data were used to calculate the molar conductance λm = (Ksol– Ksolvent) x Kcell x1000/c for all the concentrations. Where c is the concentration in moldm-3. K represents the value of observed conductance and Kcell is the cell constant. These calculated values are listed in Table 2.
Table 2: The values of η/ηo-1/c, η/ηo-1/√c , λm and α for zinc sulphate heptahydrate at 303K and 308K
S. No. |
Con |
√c (mol1/2 dm-3/2) |
303K |
308K |
||||||
η/η0 -1/c (mol-1 dm3) |
η/η0 -1/√c (mol-1/2 dm3/2) |
λm (Ω-1cm2 mol-1) |
α |
η/η0 -1/c (mol-1 dm3) |
η/η0 -1/√c (mol-1/2 dm3/2) |
λm (Ω-1cm2 mol-1) |
α |
|||
01. |
0.1 |
0.3162 |
0.2991 |
0.0946 |
65.0300 |
0.0325 |
0.5300 |
0.1676 |
59.4350 |
0.0178 |
02. |
0.2 |
0.4472 |
0.6507 |
0.2909 |
54.0150 |
0.0270 |
0.9135 |
0.4084 |
49.2675 |
0.0148 |
03. |
0.3 |
0.5477 |
0.8134 |
0.4455 |
45.3770 |
0.0227 |
0.8333 |
0.4564 |
41.1116 |
0.0123 |
04. |
0.4 |
0.6324 |
0.8575 |
0.5423 |
41.0825 |
0.0205 |
0.7813 |
0.4941 |
37.7088 |
0.0113 |
05. |
0.5 |
0.7071 |
0.8818 |
0.6236 |
33.7840 |
0.0169 |
0.8227 |
0.5817 |
30.8850 |
0.0092 |
06. |
0.6 |
0.7746 |
0.8988 |
0.6962 |
30.6533 |
0.0153 |
0.8501 |
0.6585 |
27.1542 |
0.0081 |
07. |
0.7 |
0.8366 |
0.7983 |
0.6679 |
28.5600 |
0.0143 |
0.7843 |
0.6562 |
26.2750 |
0.0079 |
08. |
0.8 |
0.8944 |
0.9399 |
0.8407 |
26.4900 |
0.0132 |
0.9291 |
0.8310 |
23.9919 |
0.0072 |
09. |
0.9 |
0.9486 |
1.0053 |
0.9537 |
25.1033 |
0.0126 |
0.9168 |
0.8697 |
22.7705 |
0.0068 |
10. |
1.0 |
1.0000 |
1.0270 |
1.0270 |
22.7940 |
0.0114 |
0.9460 |
0.9460 |
20.6145 |
0.0062 |
The variation of η/η0-1 /√c with √c follows the relationship given by the Jones-Dole equation15.
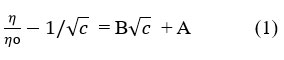
Where η, ηo and c are the viscosity of solution, viscosity of solvent and molarity of the solution respectively. A is the viscosity coefficient, which is a measure of the ion-ion or solute-solute interactions in solution and B is the viscosity coefficient which is a measure of solute-solvent or ion-solvent interaction in solution. The applicability of equation 1 has been verified by plotting the values of η/ηo – 1/√c as a function of √c by using the experimental data given in Table 2. The plots are shown in Figure 1(a) and Figure 1(b) at temperature 303K and 308K respectively.
![]() |
Figure 1: Plots between η/ηo – 1/ √c and √c for zinc sulphate heptahydrate |
It is clear from Figure 1 the plots are straight line having linearity > 0.96 which confirm the applicability of the Jones-dole equation. The value of the slope for these plots shows the presence of solute-solute interactions indicated by the positive B value (slope of the straight line). These values are given with temperature at the bottom of the plot.For both the temperatures value of A is negative indicates that ion-ion interactions are almost minimum. The positive value of B at temperature 303K (1.2773 mol-1dm3) and at temperature 308K (1.0412 mol-1dm3) suggests the structure making behaviour of zinc sulphate in water or ion-solvent interactions are present in the aqueous solution. Increase in density with increase in concentration of zinc sulphate heptahydrate indicates solvent-solvent interactions are present in solution which justify the associative nature of water molecules through hydrogen bonding. The hydration behaviour of zinc sulphate heptahydrate observed in this study on the basis of B coefficient is in good agreement with the previously reported values which are presented in Table 3.
Table 3: B and A Coefficients of aqueous zinc sulphate solutions at different temperatures
S. No. |
Jones-Dole Parameters |
Nature and magnitude of A and B coefficients |
|||||
298.15K |
303.15K |
308.15 |
313.15K |
318.15K |
Reference |
||
ZnSO4.7H2O |
|||||||
01 |
B (mol-1l) |
+0.342 |
+0.333 |
+0.320 |
+0.309 |
+0.297 |
[16] |
– |
+0.667 |
+0.676 |
0.700 |
+0.718 |
[17] |
||
– |
+0.315(±0.001) |
– |
– |
– |
[3] |
||
02 |
A (mol-1/2 l1/2) |
+10.22 |
+12.22 |
+14.12 |
+16.06 |
+17.93 |
[16] |
– |
-0.341 |
-1.191 |
-2.382 |
-3.516 |
[17] |
||
– |
+0.125(±0.0002) |
– |
– |
– |
[3] |
||
ZnSO4 |
|||||||
03 |
B (mol-1l) |
+0.339 |
+0.352 |
+0.368 |
+0.380 |
+0.393 |
[16] |
04 |
A (mol-1/2 l1/2) |
+8.59 |
+6.96 |
+4.90 |
+3.10 |
+1.05 |
[16] |
It is important that the value of dB/dT have negative sign which shows the structure maker character of the zinc sulphate in water. In the light of the hydration of the solute which undergoes a strong hydration are Kosmotropes (Structure maker) and one which undergo weak hydration are known as Chotropas (Structure breaker)18-19. The value of A has negative sign in our study which has considerable difference from the results obtained by various workers. It is one of the reasons that concentration ranges investigated by different workers. Secondly the accuracy of the measurement in the viscosities can results the variation in a value within certain limit. The nature of hydration shown by the viscosity data in the study further verified from the experimental molecular conductivity values given in Table 2. The values given in Table 2 were fitted in Kraus-Bray equation20.
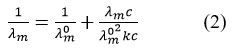
Where λm0 is the molar conductance at infinite dilution, c is the concentration and Kc is the dissociation constant. The applicability of the equation 2 has been tested by using λm values and plotting graph λmC versus 1/ λm . These plots are shown in Figure 2(a) and Figure 2(b) at temperature 303K and 308K for the aqueous solution of zinc sulphate heptahydrate in the concentration range 0.1moldm-3 to 1.0 moldm-3.
![]() |
Figure 2: Plot between λmc and 1/λm for zinc sulphate heptahydrate |
Thevalue of λm0 has been obtained from the reciprocal of intercept of the plots λmC versus 1/ λm at both the temperature. These values are given with temperature at the bottom of the plot in Figure 2. The λm0 values have been used to calculate the degree of dissociation determined by conductance ratio ( λm /λm0 ) where λm0 is the molar conductance at infinite dilution. The value of degree of dissociated are listed in Table 2. Thedegree of dissociation decreased with the increase in concentration of the salt. The variation of degree of dissociation as a function of concentration has been shown in Figure 3(a) and Figure 3(b) at temperature 303K and 308K respectively.
![]() |
Figure 3: Plot between α and c for zinc sulphate heptahydrate |
Thedegree of dissociation decreased with the increase in concentration. It is only possible that solute-solvent interaction is more stronger at higher temperature. It also implies that with the increase in concentration the degree of dissociation also decreases. The behaviour of hydration of zinc sulphate is supported by λm0 values which is directly proportional to the ion-solvent interaction and can be taken as measure of solute-solvent interaction.
On the basis of above discussion based on the viscosity and conductivity data of aqueous zinc sulphate solution. The hydration behaviour of zinc sulphate can be given by the Scheme 1.
![]() |
Scheme 1: Hydration process of zinc sulphate heptahydrate |
Ionic solid zinc sulphate heptahydrate undergo hydration in polar water through the ion-dipolar attraction between the ions and water molecules. There exist interactions between zinc ions, sulphate ions and water molecules. Hydrated metal ions or hydrated ions are surrounded by a covered shell of water molecule. The formation of ion-pairs involves in the process through electrostatic interactions along with steric effect. The decrease in is attributed due to the formation of non-conducting ion pairs21. Water crystalline seven molecule of water also participate in hydration process by dissociation in hydronium ion and hydroxyl ions which propagate the hydrogen bonding chain in the self-associated water medium. The viscometric and conductometric data have shown that hydrophilic interactions are more dominant in the hydration process because hydrophilic-hydrophilic and ion-hydrophilic interactions are responsible for solute-solvent interactions22. The viscosity data obtained in the study shows that there are three sub-concentration regions in the experimental concentration range 0.1 moldm-3 to 1.0 moldm-3. In whole concentration region viscosity of the solution followed different pattern assessed by the linearity of the plot between concentration of the salt and η/ηo-1/c For both the temperatures the plots are shown in Figure 4(a) and 4(b) at temperature 303K and 308K respectively.
![]() |
Figure 4: Plots between η/ηo – 1/c and c for zinc sulphate heptahydrate |
Although the viscosity of solution increased with the concentration of the salt as observed in the plots 4(a) and 4(b) but the magnitude of interactions do not follow the regular pattern in the whole concentration region. The process of hydration of the ions follow different pattern depending upon the nature of interactions. The different concentration regions have been assessed from the magnitude of linearity coefficient of the plot between η/ηo-1/c and c in different regions. At temperature 303K the three regions of concentrations 0.1 moldm-3 to 0.3 moldm-3 (R2=0.95), 0.3 moldm-3 to 0.6 moldm-3 (R2=0.95) and 0.7 moldm-3 to 1.0 moldm-3 (R2=0.89) exist in the aqueous solution of the salt. Similarly at temperature 308K, the three regions of concentration are 0.2 moldm-3to 0.4 moldm-3 (R2= 0.98), 0.4 moldm-3 to 0.6 moldm-3 (R2= 0.98) and 0.7 moldm-3 to 1.0 moldm-3 (R2= 0.67). The linearity coefficients of
all three concentration regions suggests that at low concentration all interactions are negligible and both the ions furnished by salt can move without any hindrance or friction. On increasing the concentration, the formation of significant tightness in the hydration cell takes place due to the interaction of water molecules with unionized and ionized species present in the solution whereas at higher concentration formation of ion-pairs by the undissociated and dissociated ions, surrounded by water molecule. The conductivity data and effect of temperature also supported the existence of three sub-concentration regions investigated on the basis of linearity coefficients of the plots η/ηo – 1/c and c in the respective concentration regions. It is clear that there is a non-linear character of the plots in Figure 4(a) and 4(b) but in different subregions the points are linearly related. The linearity coefficient in different subregions is nearly one but overall linearity coefficient shown in graph are nearly equal to ½. It is mandatory that the values of intercepts in the lower concentration region are important for applying the condition concentration ―› to zero.

Theeffective hydrodynamic radii of a rigid uncharged sphere which exhibit the same hydrodynamic behaviour as a solvated molecule or ion pair bound to water of hydration influence the viscous flow. The magnitude of the η/ηo – 1/c can be obtained from both the plots given in Figure 4(a) and 4(b) at temperature 303K and 308K respectively from the significant intercept values nearly 0.3 moldm-3 lower concentration region. This value can be used to calculate the effective hydrodynamic radii r (in cm) by the equation (3) reported in literature23.
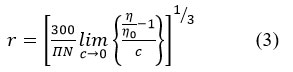
Where c is the concentration of solute in molar unit and N is the Avogadro number. The values of hydrodynamic radii of zinc sulphate(solute) in hydrated state have been calculated taking the condition of very low concentration. The values of hydrodynamic radii (r) calculated from equation 3 along with the values of degree of dissociation (αo) and molar conductivity (λm0) for both the temperatures are given in Table 4.
Table 4: The values of Hydrodynamic radii (r), degree of dissociation (αo), molar conductivity at infinite dilution ( λm0), and dissociation constant (Kc) at 303K and 308K
Temperature (K) |
Hydrodynamic radii(r) |
Degree of dissociation (αo) |
Molar conductivity at infinite dilution λm0 |
Dissociation Constant(Kc) |
303K |
2.3 Å |
0.0306 |
2000.0 Ω-1cm2mol-1 |
0.00015 |
308K |
1.7 Å |
0.0168 |
3333.3 Ω-1cm2mol-1 |
0.00004 |
The above values of hydrodynamic radii, degree of dissociation and molar conductance at infinite dilution are an indication of strong heteromolecular interaction present in the aqueous solution of zinc sulphate heptahydrate which are resultant of specific interactions namely, molecular association, charge transfer, hydrogen bonding, dipole-dipole and dipole-induced dipole interactions. In the study we have taken the concentration without considering the activity of ions. The existence of negligible of ionic strength is assumed for solute-solvent interactions in lower concentration which may be more significant for ion-ion interactions in higher concentration of the solution. The present viscometric and conductometric study on zinc sulphate heptahydrate in aqueous solution explain the hydration behaviour by the formation of ion-pair in of the solute species under experimental condition concentration and temperature. In addition the ion pairing depends on the degree of dissociation, self-association of water molecules, columbic interactions, viscosity parameters and hydrodynamic radii of the hydrated solute species.
Conclusion
The viscosity and conductance data as a function of molarity of are measured for aqueous solution of zinc sulphate heptahydrate. The experimental values were used to understand the hydration behaviour of zinc sulphate having water of crystallization. It was found that formation of ion pair takes place through the enveloping the ions by the water molecule. In the process of hydration hydrogen bonding play an important role in the interactions like proton-anion, proton-solvent association, cation-solvent and anion-solvent molecule. The values of degree of dissociation (αo), and molecular conductivity at infinite dilution supports the fact that in the process of hydration is favoured at higher temperature. The hydrodynamic radii and dissociation parameters are in agreement with the fact that both dissociated and undissociated solute molecules are involved in hydrophilic sphere around the solute species with electrostatic effect24. The hydration behaviour of solute is an important characteristics of the salts and compounds which can predict the role of precursors in the synthesis of many products including nanoparticles25. The study can be used as a model for further investigations on the intermolecular interactions and hydration behaviour of other solutes using viscometric and conductometric measurements.
Acknowledgement
The authors are thankful to Prof. R. N. Mehrotra, former Professor and Head, chemistry Department, Jodhpur University, Jodhpur (India) for help and suggestion.
Conflicts of Interest
The authors declare no conflict of interest.
Funding Sources
The author(s) received no financial support for the research, authorship, and/or publication of this article.
References
- Kant, S.; Kumar, K. J. Ind. Chem. Soc. 2008,85, 1093-1101
- Kant, S.; Dogra, P.; Kumar, S.; Ind. J. Chem. 2004, 43A, 2555-2557
- Parmar, M.L.; Thakur, R.C.; Ind. J. Chem. 2006, 45A, 1631-1637
- Vielma, T.; Kyyny, P.U.; Salminen, J.; Alopaeus, V.; Lassi, U.; J. Chem. Eng. Data 2021, 66(1), 45-57
CrossRef - Hnedkovsky, L.; Rasanen, L.; Koukkari, P.; Hefter, G.; J. Chem. Eng. Data 2021, 66(1), 38-44
CrossRef - Ammar, S.; Fievet, F.; Nanomaterials 2020, 10(1217) 1-8
CrossRef - Poul, L.; Jouini N.; Fievet, F.; Chem. Mat. 2000, 12, 3123-3132
CrossRef - Shcherbakov, V.V.; Artemkina, Y.M.; Irina; Akimova, A.; Artemkina, I. M.; Materials 2021, 14(5617), 1-26
CrossRef - Mathpal, R.; Joshi, B.K.; Joshi, S.; Kandpal, N.D.; Monatsh. Chem 2006, 137, 375-379
CrossRef - Kandpal, N.D.; Joshi, B.K.; Phys. Chem. liq. 2009, 47(3), 250-258
CrossRef - Shakeel, M.; Mehmood, K.; J. Chin. Chem. Soc. 2020, 67(9), 1552-1562
CrossRef - Loshali, R.; Kandpal, N.D.; Ind. J. Chem. 2016, 55(A), 1080-1083
- Kandpal, K.; Joshi B.K.; Kandpal, N.D.; E-J. Chem. 2007, 4(4), 574-580
CrossRef - Aneja, A.; Loshali, R.; Tamta, K.; Prasad J.; Kandpal, N.D.; Int. J. Res. Engin. Sci. 2024, 12(9), 1-8
- Jones, G.; Dole, M.; J. Ind. Chem. Soc. 1929, 51(10), 2950-2964
CrossRef - Parmar, M.L.; Sharma, P.; J. Ind. Chem. Soc. 2008, 85, 1146-1147
- Kant, S.; Kumar, S.; Thakur, M.; Arch. App. Sci. Res. 2012, 4(3), 1462-1469
- Deosarkar, S.D.; Arsule, A.D.; Sawale, R.T.; Pingle, V.G.; J. Mol. Liq. 2021, 323, 114925
CrossRef - Kant, S.; Kumar, A.; Kumar, S.; J. Mol. Liq. 2009, 150(1-3), 39 -42
CrossRef - Kraus, C.A.; Bray, W.C.; J. Am. Chem. Soc. 1913, 35(10), 1315 -1434
CrossRef - El-Hammamy, N.H.; El-kholy, M.M.; Amira, M.F.; J. Ind. Chem. Soc. 2008, 85, 1112-1115
CrossRef - Chauhan, S.; Sharma, K.; Kumar, K.; Kumar, G.; J. Sur. Det. 2013, 17, 161-168
CrossRef - Schultz, S.G.; Solomon, A.K.; J. Gen. Physio. 1961, 44, 1189-1199
CrossRef - Millero, F.J.; Chem. Rev. 1971, 71(2), 147-176
CrossRef - Mayekar, J.; Dhar, V.; Radha, S.; Int. J. Res. Eng. Tech. 2014, 03(03), 43-45
This work is licensed under a Creative Commons Attribution 4.0 International License.