Study of Photogalvanic Effect by Using of Marigold Flower as Natural Photosensitizer, Xylose as Reductant and Brij -35 as Surfactant for Solar Radiation Conversion and Storage
Shantanu Mishra* and Sushil Kumar Yadav
Solar photochemistry research lab, Department of Chemistry, Govt. Dungar College, M.G.S. University, Bikaner, Rajasthan, India
Corresponding Author E-mail: shantanuphdscholar@gmail.com
DOI : http://dx.doi.org/10.13005/ojc/400421
Article Received on : 13 Apr 2024
Article Accepted on : 22 Jul 2024
Article Published : 25 Jul 2024
Reviewed by: Dr. Shahla Danesh
Second Review by: Dr. Naresh Batham
Final Approval by: Dr. B .K Sharma
A study work plan has been put up for methodical work in the field of solar energy photogalvanic (PG) cells. It was suggested and required to do experiments with PG cells in sunlight conditions. Improving the conversion of solar energy (SE) into electricity and storing it in PG cell is the goal of our study. Many characteristics of a PG cell with an MG+D-Xylose+Brij-35 system was investigated. The open circuit voltage (Voc), voltage at dark, photopotential (PP), and photocurrent (PC) recorded in this investigation are 1076.00 mV, 163.00 mV, 913.00 mV, and 673.00 µA, respectively. Through the adjustment of PG cells' numerous parameters, the effects of solar energy were investigated. Based on the aforementioned results, surfactants (Brij-35) have demonstrated through experimentation that they are an effective system and should be further investigated, particularly with regard to improving solar energy output and storage.
KEYWORDS:Brij-35; D-Xylose; fill factor; Marigold; photocurrent
Download this article as:
Copy the following to cite this article: Mishra S, Yadav S. K. Study of Photogalvanic Effect by Using of Marigold Flower as Natural Photosensitizer, Xylose as Reductant and Brij -35 as Surfactant for Solar Radiation Conversion and Storage. Orient J Chem 2024;40(4). |
Copy the following to cite this URL: Mishra S, Yadav S. K. Study of Photogalvanic Effect by Using of Marigold Flower as Natural Photosensitizer, Xylose as Reductant and Brij -35 as Surfactant for Solar Radiation Conversion and Storage. Orient J Chem 2024;40(4). Available from: https://bit.ly/3WhMdai |
Introduction
The rapid depletion of fossil energy resources and resultantly need of alternative sources of energy are major concerns. As a result, renewable energy (RE) sources are becoming more popular, with SE being the best-looking in the future. Enhancing photogalvanics electrical output in the sphere of solar energy is the goal of the study 1. A systematic analysis of experimentally, solar parameters of photogalvanics has been studied for the D-R-S (Dye – Reductant – Surfactant) system 2. A study work plan has been put up for methodical work in the field of solar energy Photogalvanic (PG) cells3. Photogalvanic effect was studied in a photogalvanic cell containing methylene blue-xylose-NaLS+Tween-80 system4. The comparative performance of PG cells was studied for conversion and storage of solar energy by using (NaLS+Tween-80) and (NaLS+ CTAB) as different mixed surfactant with D-Xylose as reductant and Methylene blue as photosensitizer in the different mixed surfactants systems5. Later on, Ram et al. studied good results in PG cells6. Methylene blue as photosensitizer, xylose as reductant and mixed surfactant (NaLS+CTAB) were used in PG system7, Study of KOH as alkali for enhancing performance of PG cell in transparent cylindrical cell design for innovative results8. Gangotri and Lal 9, Jayshree et al. 10, Lal and Gangotri 11, Genwa and Chouhan 12, Gangotri and Lal 13, Gangotri and Meena 14, Parmila and Gangotri 15, Genwa and Genwa 16 studied PG cells. The relevant work to PG cell as provides a route for simultaneous solar power and storage 17. The dye-based study by Yadav et al. 18, PG cell with good results by Yadav and Lal 19, synthetic dyes and natural photosensitizer by Koli20 have been done for finding the relatively better and efficient dye for enhancement performance of a PG cell. For ecofriendly nature, natural dye as rose extract by Yadav21 have been reported the PG cell. Mall et al. studies on PG cell with better electrical outputs22, Lal and Gangotri studies on mixed surfactant system for PG cell 23. In addition, Jayshree et al. investigated the use of a single surfactant, a photosensitizer, and a reductant, in PG cells24. Novelty in forward-thinking research on photo-galvanic system-based prospective energy source relevant to Lal and Gangotri’s work25. Lal and Gangotri have also been working on innovative research on energy sources using a combined PG system for green environments26.
Experimental Method
Methodology and Laboratory work
An H-shaped PG cell glass tube with two arms was manufactured using the specially developed PGS (Figure 1). With the exception of a window, both arms were fully blackened and attached to a saturated calomel electrode and PT electrode, respectively. To make 25.00 ml, an H-shaped container was filled with the solutions of Brij-35, D-xylose and natural dye as MG extract, 1M sodium hydroxide, and distilled water. To measure energy output during the experiment, resistance key, carbon pot, digital pH meter, and micro-ampere were attached to both ends of the electrodes. The bulb (A 200 W filament) was used for source of PG cell. In order to set up the experiment, IR radiations were blocked using the water filter. Double-distilled water was used to prepare these solutions in order to ensure accurate electrical results. By using a titration procedure with an oxalic acid solution, sodium hydroxide solutions were standardized. To keep these solutions safe from light, they were kept in amber-colored containers. The methodology is reported using the setup shown in Figure 1 (Lal and Gangotri, 2023; Rathore et al., 2022).
![]() |
Figure 1: PG cell methodology setup |
Results And Discussion
MG variation as rose extract
For this particular photogalvanic system, a dye concentration of 5 ml is ideal (Table 1). In the course of the experiment, it was discovered that in the PG cell, the electrical results increased and reached their optimal nature at 5 ml of MG dye before decreasing (from 6 ml to 10 ml) when the resulting volume of MG dye was increased from 2 ml to 5 ml. minimum volume of MG dye (between 2 and 5 milliliters) prevent the light source from being fully absorbed, resulting in minimal electrical output. At a higher concentration range of MG (5ml), there are so many molecules that the molecule close to the electrode is not illuminated by the intended light source (Lal and Gangotri, 2023) Better outcomes were attained when the ideal molecules were present at a middle MG (5 ml) range, where the ideal source of light did reach near to the molecule. In relation to the Voc, voltage at dark, PP, and PC, the observed optimum values are 1076.00 mV, 163.00 mV, 913.00 mV, and 673 µA, in that order. The FF and CE that were observed were 1.891% and 0.2732, respectively. The observed results for the fluctuation in PG cell are shown in Table 1 and Figure 2.
Table 1: Variations of Marigold flower as natural photosensitizer, xylose as reductant and Brij -35 as surfactant
Volume/ concentration |
PP (mV) |
PC (mA) |
Power (µW) |
MG Light Intensity = 10.4 mWcm-2 Temperature = 303 K, Pt area= 1.0 cm × 0.85 cm |
|||
3.00 |
734.21 |
438.23 |
321.49 |
5.00 |
913.00 |
673.00 |
614.63 |
6.00 |
678.00 |
456.00 |
309.16 |
[D-Xylose] × 10-4 M |
|||
4.5 |
733.45 |
432.55 |
316.65 |
5.00 |
913.00 |
673.00 |
614.63 |
5.5 |
701.00 |
458.00 |
321.05 |
[Brij-35 × 10-4 M] |
|||
6.10 |
754.00 |
404.00 |
304.61 |
6.30 |
913.00 |
673.00 |
614.63 |
6.50 |
703.00 |
401.00 |
281.90 |
PH |
|||
12.20 |
654.00 |
419.00 |
274.02 |
12.30 |
913.00 |
673.00 |
614.63 |
12.40 |
703.00 |
304.00 |
213.71 |
D-xylose Variation
For this photogalvanic system, the ideal reductant concentration is 2.00 x10-4 M D-xylose (Table 1). There are fewer reductant (D-xylose) molecules are fully present to donate electrons to MB in order to produce the cationic form at lower concentrations of 1.60×10-4 M. However, when D-xylose reductant is present in higher concentrations (2.50×10-4 M), more reductant molecules are accessible to donate electrons to dye, forming the cationic form that impedes the photosensitizer (Lal and Gangotri, 2023; Rathore et al., 2022). Good results were obtained when the reductant (D-xylose) concentration was in the intermediate range (2.10×10-4 M). This is because the ideal number of D-xylose reductant molecules is present at this concentration, creating an environment that is suitable for the leuco -or semi form of photosensitizer molecules.
In relation to the open circuit voltage, voltage at dark, photopotential, and photocurrent, the observed optimum values are 1076.00 mV, 163.00 mV, 913.00 mV, and 673 µA, in that order. FF and CE were 1.891% and 0.2732, respectively, that were noted. The reductant (D-xylose) +MG+Brij-35 system’s xylose concentration change was examined, and the results were obtained (Table 1 and Figure 2).
Brij-35 Variation
For better outcomes, ideal concentration of micelles is 6.40 x10-3 M (Table 1). During the experiment, the concentration of Brij-35 increased (around its CMC value), and reached an optimal position. It then declined when the concentration of Brij-35 increased even higher. Alternatively, more surfactant molecules are accessible CMC interactions at greater concentrations of both surfactants (Brij-35 = 9.10×10-4 M), which may result in less electron transfer. The photogalvanic system’s electrical output is significantly affected at the intermediate range of surfactant concentration (Brij-35 = 9.00×10-4 M). The reason for this is that surfactants can aid in the separation of photoproducts by interacting with the micelle interface in a hydrophilic–hydrophobic manner. Based on a published study, it can be stated how the surfactants affect the system’s performance since Brij-35 has a solubilizing impact on dye.
According to a study, the active reagent like micelles improves the dye’s solubility to some degree above its CMC (Lal and Gangotri, 2023). Because a certain surfactant increases the solubility and stabilization of dye molecules in water, the electrical output generally increases when it is present. For mixes, the propensity of individual components to disperse among an unaggregated state and a combined state can differ. then only factors that could affect the processes that are important are the composition of the aggregate or the monomer. In relation to the open circuit voltage, voltage at dark, PP, and PC, the observed optimum values are 1076.00 mV, 163.00 mV, 913.00 mV, and 673 µA, in that order. The FF and CE that were observed were 1.891% and 0.2732, respectively. Table 1 and Figure 2 present the observed results for the varying levels of MG intensity in the PG cell.
Diffusion length (DL) variation
At initial rate of PC production of PG cells with the cell’s current parameter (imax, ieq) were detected with changes in DLs. Its initial rate discovered that while the equilibrium PC (ieq) exhibits very slight decreasing tendencies, the peak photocurrent (imax). Thus, in a virtual sense, the change in DL cannot affect it. The photocurrent is acquired as the minimal value and the beginning production of photocurrent were obtained on a short diffusing length (3.6 cm), where the absorbing capacity of the light source is limited by the smallest volume.
Table 2: Diffusion length’s impact on the PG system
The parameters are as follows: [D-Xylose] = 2.0 × 10-4 M, pH = 13.00, Pt electrode area = 1.0 cm × 0.85 cm, Temperature = 303 K, light intensity = 10.4 mW cm-2, and [Brij-35] = 9.0 × 10-4 M.
|
|||
DL (mm) |
Maximum PC imax (mA) |
Equilibrium PC ieq (mA) |
Rate of initial generation of photocurrent (mA min-1) |
36.0 |
768.0 |
740.0 |
17.51 |
40.0 |
913.0 |
673.0 |
61.63 |
44.0 |
986.0 |
435.0 |
16.73 |
Electrode area (EA) Variation
The maximum PC (imax) and equilibrium PC (ieq) of the photovoltaic cell (PG) with PG system were found to be regularly increasing. However, the state of equilibrium PC was practically unaffected by an increase in EA, affecting it about opposite direction. The PG system’s observed EA variation results were provided (Table 3).
Table 3: System impact of EA on PG cell.
The parameters are as follows: [D-Xylose] = 2.0 × 10-4 M, pH = 13.00, Pt electrode area = 1.0 cm × 0.85 cm, Temperature = 303 K, light intensity = 10.4 mW cm-2, and [Brij-35] = 9.0 × 10-4 M.
|
|||||
PG System |
Electrode Area (cm2) |
||||
0.75 |
0.80 |
0.85 |
0.90 |
0.95 |
|
Maximum PC imax (mA) |
462 |
566 |
711 |
674 |
388 |
Equilibrium PC ieq (mA) |
342 |
531 |
643 |
533 |
412 |
i-V characteristics of cell
Electrical circuit was used to test the short circuit current (ISC) in cell system. It was noted that the measurements of photocurrent and photopotential Vpp reached their maximum values. The isc, Voc, Vpp, and ipp—were utilized in the method to find the system’s power point and to calculate the (FF) of the cell.
The results showed that the FF and pp of the cell were, respectively, 0.2732 and 198.00 µW. For PG cell the FF was computed using equation 1.
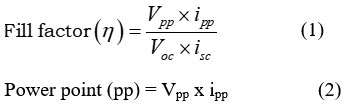
Where: Potential is expressed as Vpp, current at power point as ipp, open circuit voltage as Voc, and short circuit current as isc.
CP and CE of PG cell
The storage capacity of this PG system has proven to be good. Cell storage capacity has been investigated under specific external stress conditions. The CP was expressed in terms of t1/2. The CP of PG cells is measured in terms of t1/2, and 121.00 minutes in the dark was the observed value. Equation 2 was used to calculate the PG cell CE, which came out to be 1.891 percent. In this PG system, the PG cell’s performance and conversion efficiency (CF) were reported (Table 4 and Figure 2).

Where: Vpp stands for photopotential, ipp for photocurrent, and A for the cell’s electrode area at the power point.
Table 4: Effect of surfactants [Brij-35] with natural dye system for PG cell
S.No. |
Parameters |
Results |
1. |
Open Circuit Voltage (VOC) |
1076.00 mV |
2, |
V dark |
163.00 mV |
3. |
Photopotential (DV) |
913.00 mV |
4. |
Short Circuit Current (isc) |
673.0 mA |
5. |
Current at Power Point (ipp) |
363.0 mA |
6. |
Potential at Power Point (Vpp) |
546.0 mV |
7. |
Power at Power Point (PP) |
198.00 µW |
9. |
FF (h) |
0.2732 |
10. |
CE (%) |
1.891 % |
11. |
t1/2 |
121.0 min. |
12 |
CT (min.) |
90.0 min. |
![]() |
Figure 2: Performance of the PG Cell |
Reaction Mechanism
The ensuing chemical change occurs experimentally and shows that current is flowing electrons.
Illuminate Chamber
Excitement of MG rose molecules (as dye) caused MG to receive an electron and migrate to xylose.

Photochemical reaction at Pt electrode
The MG (dye) leuco/semi form transforms back into the initial path after cyclic shift of an electron to the electrode.

Photochemical reaction at dark Chamber:
At counter electrode

Where: MG stands for Merigold (dye), MG* for its excited form, MG– for its semi-or leuco-form, R+ is the reductant’s oxidized form, and R is the reductant (D-xylose).
![]() |
Figure 3: Brij-35-induced variations in photopotential, photocurrent, and power |
Conclusion
By choosing of appropriate redox pair in accordance with a sustainable environment, the PG cells significantly contributed to the decrease in costs necessary to achieve commercial viability. Many characteristics of a PG cell was investigated. The open circuit voltage, voltage at dark, PP, and PC recorded in this investigation are 1076.00 mV, 163.00 mV, 913.00 mV, and 673 µA, respectively. The FF and CE that are calculated were 1.891% and 0.2732, respectively. Through the adjustment of PG cells’ numerous parameters, the effects of solar energy were investigated. Based on the aforementioned results, surfactants (Brij-35) have demonstrated through experimentation that they are an effective system and should be further investigated, particularly with regard to improving solar energy output and storage.
Nomenclature
SE is Solar energy, RE is Renewable energy,PG is photogalvanic, t1/2=cell storage capacity, CT is charging time, imax is the maximal photocurrent, PGS is the photogalvanic system, DL is Diffusion length, and MG is Merigold, FF= fill factor, CE= conversion efficiency, CP = cell performance.
Highlights
Photogalvanic research is an emerging area of study.
A photogalvanic cell that is specifically designed to function better was developed.
Brings down the price of the photogalvanic cell to make it commercially viable.
The manuscript has a significant amount of storage, conversion efficiency, and electrical output.
In order to feed everyone on the planet, the scientific community worldwide is obligated to find sustainable energy sources.
Acknowledgement
The head of the chemistry department of Govt. Dungar College, M.G.S. University, Bikaner (Rajasthan), India, is appreciated by all of these authors for the laboratory facilities.
Conflict of Interest
None of the researchers have any competing interests.
References
- Meena, J.; Meena, SK.; Gangotri, KM., Orient J Chem., 2024, 40(1), 288-293.
CrossRef - Genwa, KR.; Prasad, H., Orient J Chem., 2023, 39(2), 372-377.
CrossRef - Mishra, S.; Yadav, SK., Ind. J. Sci. Tech., 2024, 17(22), 2263-2270.
CrossRef - Mohan, L.; Gangotri, KM., Res. J. Recent. Sci., 2013, 2(ISC-2012), 76-81.
- Lal, M.; Gangotri, KM., Res. J. Recent. Sci., 2013, 2(12), 19-27.
- Ram, B.; Prasad, H.; Rajoriya, V.; Genwa, KR., Ind. J. Sci. Tech., 2024, 17(19), 1914-1922.
CrossRef - Gangotri, KM.; Lal, M. Res. J. Chem. Sci., 2013, 3(3), 20-25.
- Koli, P.; Dheerata., Heliyon, 2024, 10(11) e32163. E32163.
CrossRef - Gangotri, KM.; Lal, C., Int. J. En. Res, 2000, 24(4), 365- 371.
CrossRef - Gangotri, KM.; Lal, C., J. Pow. En., 2005, 219(5), 315-320.
CrossRef - Gangotri, KM.; Meena, J., Int. J. En. Res., 2006, 28(8), 771-777.
CrossRef - Pramila, S.; Gangotri, KM., Energy Sources, 2007, 29, 1253 – 1257.
CrossRef - Genwa, KR.; Genwa, M., Solar En. Mate. Solar Cells, 2008, 92,5, 522-529.
CrossRef - Koli, P., Environmental Challenges, 2024, 14,100872
CrossRef - Yadav, SK.; Singh, G.; Yadav, RD., Int. J. Chem. Sci.,2010, 67(550), 1740-1756.
- Yadav, S.; Lal, C., En. Con. Manag., 2013, 66, 271-276.
CrossRef - Koli, P., RSC Advances, 2014, 4(86), 46194-46202.
CrossRef - Yadav, S., AIP Conference Proceedings, 2018, 1953, 080033.
- Mall, C.; Tiwari, S.; Solanki, PP., Sol. En., 2019, 55(1), 18-29.
CrossRef - Mohan, L.; Gangotri, KM., J. Solar Energy Res., 2022, 7(3),1095-1103.
- Jayshree, R.; Kumar, RK.; Pratibha, S.; Mohan, L., Res. J. Chem. Environ., 2022, 26(06), 24-29.
CrossRef - Lal, M.; Gangotri, KM., Int J Energy Res., 2022; 46(14),19538-19547.
CrossRef - Lal, M.; Gangotri, KM., Environ Sci Pollut Res., 2023,30(44):98805–98813.
CrossRef
This work is licensed under a Creative Commons Attribution 4.0 International License.