Effect of Ag ion Implantation on the Crystallinity and Optical Property of Spin-coated TiO2 Thin Films
Sunil Kumar*, Hansraj Sharma
, Jagdish Parsad
, Jagavendra Yadav
and Mangej Singh
Department of Physics, University of Rajasthan, Jaipur (India).
Corresponding Author E-mail: sunilnehra161196@gmail.com
DOI : http://dx.doi.org/10.13005/ojc/390419
Article Received on : 12 Jul 2023
Article Accepted on : 12 Aug 2023
Article Published : 24 Aug 2023
Reviewed by: Dr. Angyang
Second Review by: Dr. Neelam Sharma
Final Approval by: Dr. Ioana Stanciu
By using the spin coating method, Ag ion implanted TiO2 layers were deposited. These thin films were made on a glass substrate that had been meticulously cleaned. The characterization of synthesized Ag-dopped TiO2 thin films by XRD, UV-Vis spectrometer, and SEM with EDX. The outcomes demonstrated the presence of crystalline anatase phase and smooth surface morphologies in the Ag-TiO2 films that were annealed at 5000C. SEM results are utilized to investigate the surface morphology and element identification that has been verified by EDX analysis. The band gap for undoped TiO2 and silver-dopped TiO2 thin films is suggested by the UV-Vis investigation and we get it 2.88eV, 2.96eV, and 3.16eV subsequently. In addition, the findings showed that compared to undoped TiO2 films, the Ag-doped TiO2 film demonstrated higher photocatalytic activity.
KEYWORDS:Annealing temperature; Titanium oxide; silver doped titanium dioxide; sol-gel procedure; X-ray diffraction
Download this article as:
Copy the following to cite this article: Kumar S, Sharma H, Parsad J, Yadav J, Singh M. Effect of Ag ion Implantation on the Crystallinity and Optical Property of Spin-coated TiO2 Thin Films. Orient J Chem 2023;39(4). |
Copy the following to cite this URL: Kumar S, Sharma H, Parsad J, Yadav J, Singh M. Effect of Ag ion Implantation on the Crystallinity and Optical Property of Spin-coated TiO2 Thin Films. Orient J Chem 2023;39(4). Available from: https://bit.ly/3OFZuFO |
Introduction
Metal oxide-based semiconductors are classified as a key class of substance because of their extraordinary basic mechanical, chemical, electrical, optical, and luminous capabilities 1,2. The ability of nanosized TiO2 to act as a photocatalyst was initially identified in 1967 by Akira Fuji Shima, who then published his findings in 1972 3. This is primarily a result of its advantageous characteristics, such as non-toxicity, molecular inertness, and persistence across a broad range of pH values under illumination circumstances 4. TiO2, a semiconductor material, is utilized in a few processes, according to studies, TiO2 can be utilized in many different applications, such as dielectric layers, solar cells, self-cleaning windows, biohybrid interfaces lithium batteries, optoelectronics devices, gas detection, and air-water purification 5-11.
Due to present environmental issues, TiO2 has emerged as the prime popular semiconductor for heterogenous photocatalysis and has generated significant interest from scientists. Anatase-rutile-brookite are the three phases of TiO2 crystallization that are most often known. As a phase, rutile is the most stable although anatase is the most active.
With the help of UV light (wavelength of 380nm), the anatase band gap (Eg > 3.22eV) is activated, permitting electrons to move from the valence band to the conduction region12. As an outcome, photocatalytic reactions on TiO2 are constrained in their use of visible light as an excitation source 13.
The photons absorption on the surface to produce electron-hole pairs is one of the photocatalytic reaction’s most significant characteristics. The shape of the films, which affects their photocatalytic activities, significantly impacts this pair’s production 14,15. By altering the surface of semiconductor particles, the electron transport efficacy may be increased. Numerous techniques, such as the incorporation of TiO2 with noble metal ions and transition metal ions as well as the addition of nonmetal ions (C, B, S, F, Cl) 16,19, connected semiconductors systems 20, dye sensitization 21, can be used to alter the surface of the semiconductor. It was discovered that native metals including silver, gold, platinum, and palladium, when coated on a TiO2 surface, improve the photocatalytic efficiencies in a variety of ways, including by trapping electrons and preventing the reunify of Ele-hole pairs and improving the e/h pairs 22,23. Additionally, noble metals were shown to enhance visible light absorption, enabling surface electron activation by plasmon resonances 24. The alteration of the photocatalyst surface’s characteristic is another result of the noble metal addition 25.
Ag metal doping of TiO2 thin films has got a lot of focus since it has so many intriguing qualities, such as outstanding effectiveness, relatively inexpensive in comparison to other noble metals, antibacterial capabilities 26, and strong oxygen binding response27.
The preparation procedure has a substantial influence on all physical attributes and photocatalytic activity of thin films. Several techniques for preparing TiO2 thin films have been developed during the last two decades, including atmospheric pressure chemical vapor deposition (CVD)28, sputtering29, electron beam evaporation30, and sol-gel approach31.
The sol-gel spin-coating method is the best choice for creating vitreous or multilayer films on a range of substrates when excellent consistency, pureness, and homogeneity are required.
The main objective of the study was to develop silver-doped TiO2 (phase-anatase) opaque thin films backed by glass substrate by applying the sol-gel process. In addition, the impact of silver ions on the evolution of the crystalline structure, the mechanism of grain growth, and the characteristics of the optical and photocatalytic activity prior to and subsequent to surface enhancement with metallic silver were investigated as well.
Materials and methods
Chemicals
TTIP [Ti(O-i-C3H7)4], ethanol (CH3CH2OH), DI water, and HCl (Hydrochloric acid) all materials purchased from CDH Private Limited, Delhi. All the materials are AR grade, which is used to prepare TiO2 sol
Cleanup of Substrates
We cleaned substrates made of glass in isopropanol, Deionized water (DI), and acetone for a total of 30 minutes. The Glass substrates were lastly stored in Deionized water (DI water) before use.
Thin films deposition
For undoped thin films of TiO2 First, we combine 0.1 Mol of TTIP [Ti(O-i-C3 H7)4] and 25 ml of ethanol (CH3CH2OH) in a beaker. 2.30 hrs were used for stirring at fifty degrees Celsius. In order to lessen evaporation, the precursor solution was covered with an aluminum sheet carefully. Following that, remove the aluminum sheet and add 2gm ethyl-cellulose (as a binder) and continue stirring. After that, a solution consisting of 25 ml of ethanol, 0.1 mol of HCL, and 0.1 mol of DI water was added by dropper and stirred for 36 hours at 50 °C. then we obtained a light yellowish TiO2 solution.
For doped thin films, a solution containing 25 ml of ethanol, 0.1 mol of HCl, 0.1 mol of DI water, and silver nitrate (AgNO3– according to doping) was added and stirred for 36 hours at 500°C. then a light white TiO2 solution was sent to us.
The resulting mixture was then spin-coated at 1800 r.p.m. for 25 seconds onto a non-conducting glass substrate. To eliminate the solvents and other impurities, the deposited TiO2 thin films were heated to 110°C and baked for 3 minutes. After that, the baked film was heated for 90 minutes at 450°C.
Characterization Techniques
The X-Ray Diffraction technology “Panalytical X Pert Pro” using CuK radiation technology with CuK radiation was performed to figure out the crystal framework of pure, Ag ion implanted TiO2 thin films at the surrounding temperature. Scherrer’s equation is used to calculate the crystal size.
Using the Nova Nano SEM 450 and “scanning electron microscopy”, the surface morphology was studied. AFM using a Multimode Scanning Probe Microscope (Bruker) was used to examine the surface topography.
Spin-coated pure and Ag-implanted films served as samples and standards, and the spectral absorbance of TiO2 thin films was figured out using a LAMBDA-750 (version name) UV-Vis-NIR Spectrophotometer.
Results and analysis
XRD approach- Structural examination
To explore the structure of crystalline materials, XRD, a trustworthy and widely used identification method, is applied 32. After the analysis of the XRD data, plot a graph of undoped, 1% Ag-implanted and 2% Ag-implanted TiO2 thin films were shown in Figure 1A. Anatase is the most known phase of TiO2. The indices of the phases are listed in parenthesis and denoted by the letter “A”. These patterns clearly show that for an undoped TiO2 thin film, the diffraction peak is observed at 2θ = 25.430, which corresponds to the diffraction plane with Miller indices (101). While all of these peaks are associated with TiO2‘s anatase phase. By correlating these spikes with PDF cards #00-21-1272 and #00-21-1276, respectively, it was determined that the tetragonal anatase phase had formed. As a result, with a minor contribution from the rutile phase, these thin films largely frame out in the anatase phase. The fact that when 1% and 2% Ag were incorporated into TiO2 thin film, neither a new peak nor a change in peak position was created proved the stability of the anatase and rutile phases upon Ag doping. Instead of forming a chemical link with the Ag atoms in the TiO2 matrix, the Ag atoms simply exist there in nanoclusters 33,34.
![]() |
Figure 1: (1a) XR-D plot of the pure, (1b) 1% Ag doped & (1c) 2% Ag implanted TiO2 thin films |
The lattice parameters of the tetragonal phase were derived utilizing the following calculation 35;
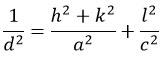
The lattice parameters, in this case, are a and c, the interplanar pitch is d, and the miller indices are (h k l).
Lattice constant measurements for undoped TiO2 are determined to be a = 3.7412 and c = 9.4676. The creation of the tetragonal phase of TiO2 was validated by such features because they matched previously published literature [PDF cards #00-21-1272 and #00-21-1276]. By adding 1% & 2% Ag to TiO2, the lattice parameters marginally grew (a = 3.74, c = 9.52), which is consistent according to the diffracted hikes relocating down to the lower 2 values.
Scherer’s formula was employed to calculate the crystallite size 36,37;
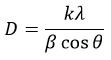
where K is the form component, with a magnitude of 0.9, λ is the CuK radiation’s wavelength, 1.55, and β is full width at half maxima. In contrast, θ provides the diffraction angle.
The size of the crystallite was measured by employing the biggest intensity XRD peak (XRD spike-101). Undoped TiO2 is found to have crystallite sizes of 35.3 nm, which are reduced to 33.03 nm and 33.96 nm when doped with 1% and 2% Ag, respectively.
Due to the deformation of the lattice and keeping in mind that the rutile phase in addition to the anatase phase of TiO2, the size of the crystallites has diminished.
Optical properties
The UV-vis transmittance plot of Ag-implanted TiO2 nanocomposite thin films is displayed in Figure 2. In the 400 nm to 900 nm wavelength range, Ag-doped TiO2 films have transmittances of more than 50%. Both the transmission and reflectance spectra’s wavy characteristics in the 800-400 nm range are caused by the interference factor, and a rapid decline in transmittance may be connected to the excitonic transition. It is clear from the somewhat undulating transmittance curve that the Ag-implanted TiO2 sheets are homogeneous and thin. The behavior of Ag-implanted TiO2 films in terms of transmittance declined as the silver concentration rose, the wavelength of the films’ intake edge migrated towards longer wavelengths and the peak’s intensity has grown 38,39. Based on the local surface plasmonic response (LSPR) effect, this type of change can be understood 40. It is a result of the addition of silver. The gap between the energy level of Ag-doped TiO2 should be less than TiO2‘s (3.2eV/3.1eV).
![]() |
Figure 2: UV-Visible absorption plot of pure and |
Band-gap
The energy difference between the valance and conduction band (Eg) is studied using the Tauc formula. The energy axis is linearly met by extrapolating (hv)1/2 v/s hv. The gap energy value is determined by this energy plot. The plot of (hv)1/2 v/s hv is shown in Figure 3. Thin films of undoped TiO2, 1% Ag, and 2% Ag doped-TiO2 have corresponding band gaps of 3.16 eV, 2.96eV, and 2.88ev. The band-gap energy is varying between 3.2eV and 2.8 eV as a result of Ag doping into TiO2. The appearance of lattice distortion brought on by silver doping, which generated fresh levels of energy levels inside the TiO2 thin film’s forbidden band, is the potential cause of this band gap reduction 41. The development of metal groupings, which resulted in regional energy levels and surface absorption, was another factor in the band gap’s reduction 42.
![]() |
Figure 3: Band-gap plot for undoped and Ag-implanted TiO2 thin films |
SEM Analysis
pure and silver-implanted TiO2 films grown by the sol-gel technique on Si substrates are depicted in SEM images in Fig. 4. The type of Ag incorporation (doping) into TiO2 film may be identified using excellent quality SEM imaging and EDX examination. SEM pictures indicate dense clusters of nano craters with sizes between 60 and 170 nm. In excellent quality SEM imaging, the particular nanoparticle dispersion both inside and around the nano fissures is visible (Fig-5).
Ag-nano-dots are nanoparticles that are around 30 nm in size when they are isolated, but they can aggregate to produce particles that are 200 nm in size. According to some research, increasing the Ag content may enhance the propensity to produce Ag-agglomerates.
There are no traces of the Ag component seen in the Silver doped TiO2 films’ EDX spectra (Fig. 5c). This was allocated to the increased dispersion of silver nanoparticles on the top area of the film, which limited EDX observation. The detected signal of underneath silver nanoparticles is in fact too tiny to be separated from the background signal when employing the standard acceleration voltages of 5 keV to 20 keV.
![]() |
Figure 4: SEM pictures showing the surface morphologies of undoped TiO2 film(a), 1% Ag-doped (b), and 2% Ag-implanted TiO2 film (c). |
![]() |
Figure 5: EDX plot of pure and Ag-doped TiO2 |
Conclusions
The relatively low-cost sol-gel method has been used to create thin films of Ag-doped TiO2 at a heating temperature of 500°C.
In comparison to pure TiO2 films, Ag-doped TiO2 films have smaller crystallite domain sizes.
According to X-ray diffractograms, TiO2 developed into the anatase phases, whose lattice parameters initially grew up with Ag doping and then decreased when the fluence value was further raised.
Compared to undoped TiO2 films, Ag-doped TiO2 films have a greater photocatalytic function. The Eg is decreased with doping.
Acknowledgments
We received funding for this study from the CSIR in New Delhi, and characterization resources came from the MNIT MRC and Manipal University in Jaipur. We appreciate the facilities for experimentation and characterization provided by USIC and the physics department at UOR, Jaipur.
Conflict of Interest
The authors declare that they have no known competing financial interests or personal relationships that could have appeared to influence the work reported in this article.
References
- Canas -Carrell J., Metal oxide nanomaterials: health and environmental effects, Health and Environmental Safety of Nanomaterials (Elsevier, Amsterdam, 2014), pp. 200–221
CrossRef - Kumar S., Metal oxides for energy applications, Colloidal Metal Oxide Nanoparticles (Elsevier, Amsterdam, 2020), pp. 471–504
CrossRef - Fujishima A., Honda K., Electrochemical photolysis of water at a semiconductor electrode. Nature 238(5358), 37–38 (1972)
CrossRef - Huang L, Sun C, Liu Y. Pt/N-codoped TiO2 nanotubes and its photocatalytic activity under visible light. Appl Surf Sci. 2007; 253(17):7029-7035.
CrossRef - Alexandrov P., Koprinarova J., Todorov D., Dielectric properties of TiO2-films reactively sputtered from Ti in an RF magnetron, Vacuum, Volume 47, Issue 11, November 1996, Pages 1333-1336
CrossRef - Dong Y. X., Wang X. L., Jin E. M., Jeong S.M. , Jin B. , Lee S. H. , One-step hydrothermal synthesis of Ag decorated TiO2 nanoparticles for dye-sensitized solar cell application, Renewable Energy, Volume 135, May 2019, Pages 1207-1212
CrossRef - Choi T., Kim J. S., Kim J. H., Transparent nitrogen doped TiO2/WO3 composite films for self-cleaning glass applications with improved photodegradation activity, Advanced Powder Technology Volume 27, Issue 2, March 2016, Pages 347-353
CrossRef - Wang J., Wang Z., Wang W., Wang Y., Hu X., Liu J., Gong X. , Miao W., Ding L. , Li X., and Tang J., Synthesis, modification and application of titanium dioxide nanoparticles: a review, Royal society of chemistry, Nanoscale, 2022, 14, 6709-6734
CrossRef - Javed H.M.S., Adnan M., Qureshi A.A., Javed S., M., Akram M.A., Shahid M., Ahmad M.I., Afzaal M., Abd-Rabboh H.S.M., Arif M., Morphological, structural, thermal and optical properties of Zn/Mg-doped TiO2 nanostructures for optoelectronic applications, Optics & Laser Technology, Volume 146, February 2022, 107566
CrossRef - Ramesan M.T. , Santhi V. , Bahuleyan B.K. , Al-Maghrabi M.A. , Structural characterization, material properties and sensor application study of in situ polymerized polypyrrole/silver doped titanium dioxide nanocomposites, Materials Chemistry and Physics, Volume 211, 1 June 2018, Pages 343-354
CrossRef - Lee S.Y., Park S.J., TiO2 photocatalyst for water treatment applications, Journal of Industrial and Engineering Chemistry, Volume 19, Issue 6, 25 November 2013, Pages 1761-1769
CrossRef - Kormann C., Bahnemann D.W., Hoffmann M.R., Preparation and Characterization of Quantum-Size Titanium Dioxide, J. Phys. Chem. 92 (1988) 5196.
CrossRef - Goswami D.Y., A review of engineering developments of aqueous phase solar photocatalytic detoxification and disinfection processes, J. Sol. Energy 119 (1997) 101.
CrossRef - Kumar K.J., Raju N.R.C., Subrahmanyam A., Thickness dependent physical and photo catalytic properties of ITO thin films prepared by reactive DC magnetron sputtering, Applied Surface Science 257 (2011) 3075.
CrossRef - Chung Y. W., Yuan L. L., Yu S.L., Chen J.L., Chien H.W., Thickness-dependent photocatalytic performance of nanocrystalline TiO2 thin films prepared by sol–gel spin coating. Applied Surface Science 280 (2013) 737.
CrossRef - Gaya UI, Abdullah AH. Heterogeneous photocatalytic degradation of organic contaminants over titanium dioxide: a review of fundamentals, progress and problems. J Photochem. Photobiol. C 9 (2008) 1.
CrossRef - Chen C., Li X., Ma W., Zhao J., Hidaka H., Serpone N., Effect of transition metal ions on the TiO2-assisted photodegradation of dyes under visible irradiation: a probe for the interfacial electron transfer process and reaction mechanism, J. Phys. Chem. B 106 (2002) 318.
CrossRef - Ko S., Photochemical synthesis and photocatalytic activity in simulated solar light of nanosized Ag doped TiO2 nanoparticle composite. Composites: Part B (2010), doi:10.1016/j. composites B, 2010.09.007
- Rosseler O., Shankar M.V., Dum K.L., Schmidlin L., Keller N., Keller V., Solar light photocatalytic hydrogen production from water over Pt and Au/TiO2 (anatase/ rutile) photocatalysts: influence of noble metal and porogen promotion. J. Catal. 269 (2010) 179.
CrossRef - Khan R, Kim T-J. Preparation and application of visible-light-responsive Ni-doped and SnO2-coupled TiO2
nanocompositephotocatalysts, J. Hazard Mater. 163 (2009) 1179.
CrossRef - Hilal H.S., Majjad L.Z., Zaatar N., El-Hamouz A., Dye-effect in TiO2 catalyzed contaminant photo-degradation: sensitization vs. charge-transfer formalism, Solid State Sciences 9 (2007) 9.
CrossRef - Thompson T.L., Yates J. J., Surface science studies of the photoactivation of TiO2-new photochemical processes, Chem. Rev. 106(10) (2006) 4428.
CrossRef - Fujishima A., Zhang X., Tryk D.A., TiO2 photocatalysis and related surface phenomena. Surf. Sci. Rep. 63 (2008) 515.
CrossRef - Kim S., Hwang S., Choi W., Visible light active platinum-ion-doped TiO2 photocatalyst, J. Phys. Chem. B 109 (2005) 24260.
CrossRef - Aprile C., Corma A., García H., Enhancement of the photocatalytic activity of TiO2 through spatial structuring and particle size control: from subnanometric to submillimetric length scale, Phys. Chem. 10 (2008) 769.
CrossRef - Sharma V.K., Yngard R.A., Lin Y., Silver nanoparticles: green synthesis and their antimicrobial activities. Adv. Colloid Interface Sci. 145 (2009) 83.
CrossRef - Liu S.X., Qu Z.P., Han X.W., Sun C.L., A mechanism for enhanced photocatalytic activity of silver-loaded titanium dioxide, Catal. Today 93 (2004) 877.
CrossRef - Jung S.C., Kim B.H., Kim S.J., Imaishi N., Cho Y.I.,Characterization of a TiO2 Photocatalyst Film Deposited by CVD and Its Photocatalytic Activity, Chemical Vapor Deposition 11 (2005) 137.
CrossRef - Damiriv D., Bally A.R., Ballif C., Homes P., Schmid P.E., Sanjines R., Levy F., Parvulescu V.I., Photocatalytic degradation of phenol by TiO2 thin films prepared by sputtering, Appl. Catal. B, 25 (2000) 83.
CrossRef - Hoyer P., Formation of Titanium Dioxide Nanotube Array, Langmuir 12 (1996) 1411.
CrossRef - Burnside S.D., Shklower V., Barbe C., Comte P., Arendse F., Brookes K., Grätzel M., Self-organization of TiO2 nanoparticles in thin films, Chem. Mater. 10 (1998) 2419.
CrossRef - Barakat NA, Kanjwal MA, Chronakis IS, Kim HY. Influence of temperature on the photodegradation process using Ag-doped TiO2 nanostructures: negative impact with the nanofibers. J Mol Catal A Chem. 2013; 366:333-340. Sakthivel S, Shankar MV, Palanichamy M, Arabindoo B,
CrossRef - Bahnemann DW, Murugesan V. Enhancement of photocatalytic activity by metal deposition: characterisation and photonic efficiency of Pt, Au and Pd deposited on TiO2 catalyst. Water Res. 2004;38(13):3001-3008
CrossRef - Bensouici F, Souier T, Dakhel AA, Iratni A, Tala-Ighil R, Bououdina M. Synthesis, characterization and photocatalytic behavior of Ag doped TiO2 thin film. Superlattices Microstruct. 2015;85: 255-265.
CrossRef - Djerdj I, Tonejc A. Structural investigations of nanocrystalline TiO2 samples. J Alloys Compd. 2006;413(1-2):159-174.
CrossRef - Khan M, Farooq WA, Saleem M, Bhatti KA, Atif M, Hanif A. Phase change, band gap energy and electrical resistivity of Mg doped TiO2 multilayer thin films for dye sensitized solar cells applications. Ceram Int. 2019;45(17):21436-21439
CrossRef - Ao Y, Xu J, Fu D, Yuan C. Preparation of Ag-doped mesoporous titania and its enhanced photocatalytic activity under UV light irradiation. J Phys Chem Solid. 2008;69(11):2660-2664.
CrossRef - Sakthivel T, Ashok Kumar K, Ramanathan R, Senthilselvan J, Jagannathan K. Silver doped TiO2 nano crystallites for dyesensitized solar cell (DSSC) applications. Mater Res Express. 2017;4(12):126310.
CrossRef - Usha K, Kumbhakar P, Mondal B. Effect of Ag-doped TiO2 thin film passive layers on the performance of photo-anodes for dye-sensitized solar cells. Mater Sci Semicond Process. 2016;43: 17-24.
CrossRef - Eagen C. Nature of the enhanced optical absorption of dyecoated Ag is land films. Appl Optics. 1981;20(17):3035-3042.
CrossRef - Demirci S, Dikici T, Yurddaskal M, Gultekin S, Toparli M, Celik E. Synthesis and characterization of Ag doped TiO2 heterojunction films and their photocatalytic performances. Apply Surf Sci. 2016; 390:591-601.
CrossRef - Li Y, Ma M, Chen W, Li L, Zen M. Preparation of Ag-doped TiO2 nanoparticles by a miniemulsion method and their photoactivity visible light illuminations. Mater Chem Phys. 2011; 129(1-2):501-505.
CrossRef
This work is licensed under a Creative Commons Attribution 4.0 International License.