Validation of Essential Oils’ Gas Chromatography Mass Spectrometry Compositional Analysis Using System independent parameters; Kovat Index and Fragmentation Pattern
Department of Chemistry, National Open University of Nigeria.
Corresponding Author E-mail: rmusa@noun.edu.ng
DOI : http://dx.doi.org/10.13005/ojc/380608
Article Received on : 18 Oct 2022
Article Accepted on :
Article Published : 08 Dec 2022
Reviewed by: Dr. Moondra Zubir
Second Review by: David .R
Final Approval by: Dr. S. A. Iqbal
One of the challenges in determination of essential oils components using GSMS is the variation of the retention time observed. Kovat index was calculated for each compound identified in eight essential oils samples while the fragmentation pattern of four compounds were illustrated. Kovat index shows, P-xylene has 809 KI, α-pinene 934 and another value of 905. In some oil samples KI value for Limonene is 938, α- Phellandrene has 932 and another value of 933 which occurred at slightly higher retention time of 6.659. Caryophyllene has the lager KI value of 1254 and is consistent in all the samples of the essential oils. The fragmentation pattern for P-xylene, α-pinene, caryophyllene and 1-octen-3-ol were illustrated. The justification of each peak in the mass spectra and the consistency in the values of Kovat index have validated the results obtained from GCMS analysis of the essential oils understudy.
KEYWORDS:Essential oils; Fragmentation pattern; Kovat index; Molecular ion
Download this article as:
Copy the following to cite this article: Runde M. Validation of Essential Oils’ Gas Chromatography Mass Spectrometry Compositional Analysis Using System independent parameters; Kovat Index and Fragmentation Pattern. Orient J Chem 2022;38(6). |
Copy the following to cite this URL: Runde M. Validation of Essential Oils’ Gas Chromatography Mass Spectrometry Compositional Analysis Using System independent parameters; Kovat Index and Fragmentation Pattern. Orient J Chem 2022;38(6). Available from: https://bit.ly/3Bl6bXQ |
Introduction
The separation, identification and quantification of compounds in a mixture when Gas chromatography is used can be generated from the values of the retention time of the eluting compound (Etxebarria, et al, 2009). Factors which can affect the retention and consequently affect the results of Gas chromatographic analysis are unstable temperature and pressure, column degradation or matrix effects (El-Naggar, 2013). For this reason, researchers have put in more efforts in order to reduce the variation arising from the factors listed above. According to Etxebarria et al, 2009, peak alignment algorithms, electronic pneumatic system and retention time locking are some futures added to the GC and they help in reducing variation by controlling the flow of the carrier gas, fixing the retention time and implementing peak deconvolution algorithms, and treating the chromatograms. In some experiments, this variation can be optimized using computer simulation having knowledge on the parameters that control the retention time (Yasar, et al, 2009). In the work presented by (Yasar, et al, 2009), retention durations for gas chromatography were foretold using thermodynamic method that made use of the two quantifiable variables entropy change (delta S) and enthalpy change (delta H). Also, an insight of compound partition parameters can be used to predict retention time of the compound.
Retention index is used in the identification of essential oils components. When retention results exist and are obtained from columns with the same charges and are compared, it is possible to report retention indices which can be conveniently utilized for identification of compounds (Jennings and Shibamoto, 1980). Retention Index in liquid chromatography refers to the period of time between the injections of an analyte band and the recording of the peak maximum (tR). The adjusted retention time (t1R) is the time from the point of injection to the peak maximum minus the time tM which is required for an un-retained component to pass through the column (t1R – tM) (Babushok and Zenkevich, 2009).
Kovat retention index
The retention time is relatively sensitive to slight changes in experimental conditions such as column changes, column length, column diameter, carrier gas, flow rate, pre-column, and more, while the retention index Kovats is more reliable and reproducible
The Kovat retention index is a continuous and standard parameter, whereas, the retention time can be affected by experimental adjustment of the system such as precolumn settings and flow rate, the column type and size, and the type of the carrier gas, (Idroes, et, al, 2019). In order to convert retention time into a more trustworthy and reproducible system, the Kovats retention index was conceptualized in to the analysis of gas chromatography. E. Kovats came out with the idea of this retention index in a gas chromatographic system experiment in 1958. A compound’s retention index is determined in relation to two reference compounds so that the instrument’s influence can be discounted and the accuracy can be raised
Retention indices are metrics that compare a specific solute’s retention information to that of a reference solute, typically utilized in n-alkane series. In the Kovats Retention Index System (KI), n-alkanes are employed as homologs (Sandra and Bicchi, 1987).

where t1 R(C) and t1R(C+c) are the modified retention duration of n-alkanes with carbon number C and C+c respectively eluting before and after solute A, with the modified retention duration t1R (A).
In actuality terms, the modified retention duration is derived by deducting the solute’s real retention from that of mixture of gasses example air or a solvent that boils at a very low temperature. Both polar and non-polar stationary phases, can be channeled to measure KI. The Kovats indices might theoretically be determined under isothermal conditions. (Sandra and Bicchi, 1987). Retention indices can be used to ascertain the order in which polar and non-polar solutes elute
Fragmentation pattern
The molecules of essential oils understudy acquire energy in the ionization and became unstable so they dissociate with the purpose of attaining stability. This process of dissociation by molecules as they travel through the ionization follow a systematic pattern peculiar to the molecule understudy, is refer to as fragmentation pattern. The knowledge of fragmentation pattern enable scientist to determine the molecular weight as well as the structure of the molecule understudy (McLafferty, 1993). The weaker Carbon to carbon bonds are likely to dissociate when interpreting fragmentation pattern of a molecule. Other factors of concern in fragmentation are discussed below:
But first note that the molecular ion peak is presented as:

This occur when the vaporized sample is bombarded by electrons in the ionization chamber and later produce an ion by removal of an electron from the parent molecule.
The unstable molecular ion would fragment in to two parts, one positive ion and a neutral neutral free radical.

STEP 1
Tertiary, secondary, and primary branched carbon atoms are more likely to undergo cleavage first, with the positive charge sticking on the branched carbon giving more stable radical called carbonium ion.
STEP 2
Cleavage occurs first where there is double bond and at the beta to the double bond.

STEP 3
A ring is frequently present in a substance with a strong molecular ion peak, and the intensity of the depends on the stability of the ring.
STEP 4
Cyclic compounds typically have peaks that correspond to the mass number such as for aromatics; C6H5+ at m/z = 77 and for alkylbenzene; C7H7+ at m/z = 91
STEP 5
The alpha carbon in a saturated ring is targeted for cleavage, and when two ring atoms are loss the intensity of the peak is higher compare to when one ring atom is loss.
STEP 6
When an alkyl-substituted cyclic compound has a double bond adjacent to the side chain, cleavage is most likely to occur at the bond beta to the ring.
STEP 7
Cleavage at the bond beta to a hetero-atom will be encouraged.
STEP 8
Keto-group-containing compounds frequently break at this group, leaving the positive charge on the carbonyl end
STEP 9
Neutral compound are likely to be lost, for example, H2O is loss from alcohol, in some instances CO or HCN are loss.
(Kennepohl, 2022).
Data from previously GCMS results obtained from my analysis of some essential oils were revisited and their parameters of Kovat indices analyzed as a way of eliminating system dependency when determining composition of essential oils using GCMS.
The Kovat indices were calculated using the formula described by Sandra and Bicchi, 1987.

The entire procedure adopted for the determination of chemical constituents of essential oils is described in the work reported by Runde et, al, 2015 and the column type is HP-5MS while helium gas was used.
Method
Gas Chromatographic Mass spectrometry (GC/MS) Analysis
Column type was HP-5ms, 5% phenyl methyl silox: 469.56 509 in the J and W Scientific Gas Chromatography system with a direct mass spectrometer linkage (model GC Agilent S/N 20102969, polaris Q S/N 210729) The following conditions were met when using capillary column (30M x 250M): Ovum temperatures ranged from 500 C for one minute to 200 C/minute to 2000 C/minute to 3000 C/minute for two minutes.
The injected sample was 0.2L of diluted oil in hexane, splitless injection methods, an electron ionization (EI) mode ion source temperature of 2300C, and an ionization energy of 70ev. Based on a comparison of the retention indices and mass spectra of the bulk of the substance with data produced under identical procedures, the constituents of the essential oils were determined.
The retention indices (RI) are in reference to a homologous sequence of n-alkanes on the GC column under the same chromatographic conditions. The relative concentration of the components was calculated using peak area normalization (Ramzi et al., 2013).
Results
Table 1: Kovats Indices (KI) of some compounds obtained from GCMS analysis of essential oil
Constituent |
RT (min) |
Area % |
KI |
MW |
Plant essential oils |
1P-xylene |
5.490 |
0.228 |
809 |
106 |
BD |
2Alpha-Pinene |
6.747 |
18.515 |
934 |
136 |
BD |
1P-Xylene |
5.484 |
0.24 |
809 |
106 |
OA |
2Alpha-Pinene |
6.715 |
2.664 |
934 |
136 |
OA |
3Copaen |
12.102 |
0.145 |
1242 |
204 |
OA |
4cis-beta Terpineol |
11.009 |
3.914 |
1020 |
152 |
OA |
2Alpha-Pinene |
5.299 |
30.536 |
905 |
136 |
HS |
5Limonene |
6.914 |
4.378 |
938 |
136 |
HS |
2Alpha-Pinene |
5.299 |
30.536 |
905 |
136 |
HSu |
5Limonene |
6.914 |
4.378 |
938 |
136 |
HSu |
6Caryophyllene |
12.698 |
3.083 |
1254 |
204 |
HSu |
2Apha .pinene |
5.264 |
10.285 |
905 |
136 |
FV |
7β-myrcene |
6.252 |
1.290 |
925 |
136 |
FV |
5Limonene |
6.911 |
1.332 |
938 |
136 |
FV |
3Copaene |
12.108 |
0.599 |
1242 |
204 |
FV |
7β-myrcene |
13.139 |
2.889 |
1062 |
136 |
FV |
8Alpha.caryophllene |
13. 214 |
11.397 |
1264 |
204 |
FV |
9β-Pinene |
5.274 |
8.073 |
905 |
136 |
EU |
9β-Pinene |
6.024 |
0.329 |
920 |
136 |
EU |
7β-myrcene |
6.265 |
0.515 |
925 |
136 |
EU |
9β-Pinene |
6.302 |
1.952 |
926 |
136 |
EU |
10alpha.-phellandrene |
6.608 |
13.990 |
932 |
136 |
EU |
113-Carene |
6.625 |
3.729 |
932 |
136 |
EU |
10alpha.-Phellandrene |
6.643 |
2.524 |
932 |
136 |
EU |
10alpha.-Phellandrene |
6.659 |
2.768 |
933 |
136 |
EU |
12Eucalyptol |
7.073 |
13.101 |
941 |
154 |
EU |
7β-myrcene |
6.252 |
0.116 |
925 |
136 |
SB |
5Limonene |
6.908 |
0.126 |
938 |
136 |
SB |
2alpha-Pinene |
7.041 |
1.897 |
940 |
136 |
SB |
13Camphene |
11.501 |
0.101 |
1030 |
136 |
SB |
14Alpha-cubebene |
11.708 |
0.050 |
1234 |
204 |
SB |
3Copaene |
12.103 |
1.600 |
1242 |
204 |
SB |
6Caryophyllene |
12.697 |
5.332 |
1254 |
204 |
SB |
6Caryophllene |
12.678 |
0.863 |
1254 |
204 |
MS |
15Guaiol |
15.648 |
5.823 |
13012 |
222 |
MS |
Key: BD (Bosweillia dalzielii), OA (Ocimum americanum), SB (Stachys byzantina), HS (Hyptis spicigera), HSu (Hyptis suaveolens), FV (Fraxinus velutina), EU (Eucaliptus comandulensis), SB (Stachys byzantina), MS (Monardella villosa)
Discussion
From the results presented in table 1, the Kovats indices for a total of 15 compounds were calculated from the previously data obtained from the GCMS analysis of eight different essential oils samples. However, these samples were analyzed using the same GCMS equipment at the same operating conditions. P-xylene were identified in two different samples of essential oils (Bosweillia dalzielii, BD and Ocimum americanum), α-pinene were identified in 6 different samples, Copaene was identified in 3 samples, Cis-β-terpeneol was identified in one sample (Table 1). Similar compounds were assigned the same superscript while the sourced parent plant from which the essential oils were extracted are abbreviated (see full names in the key). The Kovat index for p-xylene obtained from Bosweillia dalzielii (BD) and Ocimum americanum (OA) are 809. There are some similarities observed in the Kovat indices of α-pinene obtained from essential oils of Hiptis spicigera (HS), Hyptis suaveolence (HSu), and Fraxinus velutina (FV). Other samples; Bosweillia dalzielii, Ocimum americanum and Stachys byzantina varied as each have 938,934 and 940 respevtively. However, different researchers have reported different Kovat index values in a GCMS analysis of samples using different column (Hognadottir and Rouseff, 2003). Copaene is seeing to have maintained its Kovat index value at 1242 for all the samples of the essential oils from Ocimum americanum, Flaxinus velutina and Stachys byzantina. Adedeji reported value for Copaene Kovat index as 1353 (Adedeji, et al, 1993), this value is higher than what we got in our work. This variation could be as a result of different column type (DB-1) used by the author and HP-5MS use in this research. Another compound, Limonene was obtained from the essential oils from Hyptis spicigera, Hyptis suaveolense, Frexinus velutina and Stachys byzantina was consistent with Kovat index value of 938. However, when different column type is used in a GCMS analysis, it would have given a different Kovat index for Limonene as presented by Jordan, et al, 2002 where the Kovat index for Limonene is 1031 and the column type use is HP-5. Caryophyllene was present in three different samples; Hyptis suaveolense, Stachys byzantina and Monardella villosa. These samples were seeing to have presented the same values of Kovat indices of 1254. Caryophyllene has Kovat index value of 1416 (Mohammed, 2020), which is higher than what we got in our work, though the column type used was not reported by the author. The Kovat indices for β-Myrcene obtained from essential oils samples of Flaxinus velutina, Eucalyptus comandulences and Stachys byzantina are 925 following their retention time at 6.265 and 6.252 respectively. However, a different value 1062 was obtained in the same Flaxinus velutina where the retention time is 13.139 min. This implies that there are other factors affecting the value of a Kovat index aside the coulumn type of Gas chromatography. A different value of 988 was reported in the work of β-myrcene (Jirovetz, et al, 2003) while the column type used is FSOT-RSL-200. β-Pinene, an isomer of α-pinene, obtained from essential oils sample of Eucalyptus comandulences has three different Kovat indices of 905, 920 and 926. This revealed that the Kovat index value are not the same among isomers, as such it can be assumed that Molecular weight of a compound does not have significant effect on the Kovat index of that compound. α-Phellandrene has Kovat index value of 932 at retention time of 6.608, 6.625 and 6.643 while a different value of 933 was observed when the retention time increased to 6.659 minutes. Other compounds are, Eucalyptol and Carene all from Eucalyptus comandulences which have Kovat index value of 941 and 932 respectively, while Cis-β-terpeneol, Camphene and Carene obtained from the samples of Ocimum Americanum, Stachys byzantina and Monardella villosa have Kovat indices of 1020,1030 and 13012 respectively. Though the Kovat index is for the purpose of eliminating system dependency, the critical observation of our work shows that some Kovat indices for the same compounds in the same sample have some level of variation. We also observed that these variations occur when there is difference in the retention time of the same compound in the same sample or the same compound from different sample.
Fragmentation pattern of α-Pinene and P-Xylene constituents of Bosweillia dalzielii essential oils
![]() |
Figure 1: Spectrum of α-Pinene as revealed by GCMS analysis of Bosweillia dalzielii essential oils |
Fragmentation pattern of α-pinene
Alpha Pinene radical cation, a molecular ion that appeared at m/z 136 in the mass spectrum, was produced when -pinene exited the GC-column of the GC-MS instrument and entered the ionization chamber (Figure 1).

The peaks in this mass spectrum are caused by The arrival of the cations of different sizes at the detector result to the peaks in this mass spectrum. Because of internal ionization chamber fragmentation that takes place shortly after the creation of the molecular ion, peaks smaller than the molecular ion have lost a neutral fragment, such a radical.
The base peak, which has been given an arbitrary 100% abundance, can be found at m/z 93. Loss of the neutral m/z 43 fragment is to blame for this. The set of equations below expresses additional peaks that have emerged as a result of group loss.
The removal of the methyl group led to the peak at m/z 121.

The base peak, which was caused by the potential loss of the isopropyl group, lies at m/z 93..

The removal of a tertiary alkyl group led to the appearance of the peak at m/z 51

Fragmentation pattern of P-xylene
![]() |
Figure 2: Spectrum of p-Xylene as revealed by GCMS analysis of Bosweillia dalzielii essential oils |
Similar process occurs on the P-xylene compound as it exits into the ionization chamber in which an electron is knocked off from the molecule to create a molecular ion P-Xylene radical cation that shows at m/z 106 on the mass spectrum.

The cleavage of the methyl (CH3) group produced the peak at m/z 91, which is the base peak.

The removal of the ethyl group (.C2H5) was what caused the peak to occur at 77.

Fragmentation pattern of caryophyllene and 1-octen-3-ol obtained from analysis of Essential oil of Hyptis suaveolens
![]() |
Figure 3: Mass Spectrum of caryophyllene obtained from analysis of Essential oil of Hyptis suaveolens |
Fragmentation pattern of caryophyllene
The impact of the high energy electrons in the ionization chamber is what causes the molecular ion of caryophyllene to form, and the subsequent emergence of the peak at m/z 204 (Figure 2) is the result of this. The creation of a peak at m/z 189 was caused by the removal of a methyl group. The loss of the ethyl, propyl, butyl, pentyl, and hexyl groups, is represented by peaks at m/z 175, 161, 147, 133, and 105 respectively. After the base peak, the caryophyllen fragmentation continued with the loss of various groups until the loss of the least group, which led to the formation of the peak at m/z 15. The detailed fragmentation of caryophyllene is giving below.

![]() |
Figure 4: Mass Spectrum of 1-octen-3-ol obtained from analysis of Essential oil of Hyptis suaveolens |
A molecular ion was formed when the 1-octen-3-o1 molecule exited the GC-column of the GC-MS instrument and entered the ionization chamber. This molecular ion was visible at the mass spectrum’s m/z 129.
Other peaks observed resulted from the arrival of various sizes of radical cation to the detector. The base peak, which has been given an arbitrary 100% abundance, can be found at m/z 57.
Below are the processes that led to the formation of peaks during the fragmentation of 1-octen-3-ol
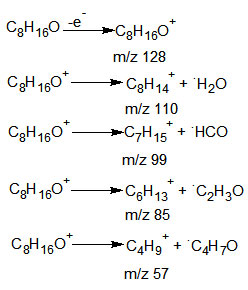
Conclusion
Essential oils are becoming necessary commodity as time goes by, as such it is critical that users should be fortified with the components of these culinary oils. As it stans, GCMS is one the reliable instrument for quantification and identification of the components of essential oils. However, this instrument is not without challenges of accuracy due to some factors ranging from variation in temperature and pressure, column degradation and so on. The basis of separation of complex mixture in to various components using GS is the differences in the retention time of each compound. In this work, similar compounds from different samples of essential oils have presented slightly different retention time, given enough reason to fall back on a more ground leveling parameter in order to confirm the existence of the compounds in both the essential oil samples. One of such parameters adopted in this work is the Kovat index and it has been successfully used to authenticate the result of the GCMS analysis of eight essential oils samples obtained earlier. Therefore, Kovat index is that leveling parameter which can be used to authenticate the results of GCMS analysis for essential oils credit to it ability to eliminate system dependency.
P-xylene, α-pinene, caryophyllene and 1-octen-3-ol are few of the components of essential oils analyzed in this work. The step-wise pattern demonstrated also validate the mass spectrum obtained. This is because it was possible to justify each peak that appeared on the spectrum. The combined approach of Kovat index and fragmentation pattern of these compounds has validated the result of our work.
Reference
- Etxebarria N., Zuloaga O., Olivares M., Bartolomé L.J. and Navarro P. Retention-time locked methods in gas chromatography. J. of Chro. A, 2009 1216, 1624–1629
CrossRef - El-Naggar A.Y. Factors affecting selection of mobile phase in gas Chromatography. Ame. J. of Re Com. 2013 1 (3), 219-228
CrossRef - Yasar T., Fredrik S. and Andres C. Retention time prediction of compounds in Grob standard mixture for apolar capillary columns in temperature-programmed gas chromatography. Anal. and Bio-anal Chem, 2009 1(393), 327-334
CrossRef - Jennings W.G. and Shibamoto T. Quantitative analysis of flavor and fragrance volatiles by glass capillary GC. Aca. Pre, New York 1980
- Babushok V.I., Zenkevich I.G. Retention Indices for Most Frequently Reported Essential Oil Compounds in GC. Chro. 2009 3(4), 257-269
CrossRef - Idroes R., Japnur A.F., Suhendra R. and Rusyana A. Kovats Retention Index analysis of flavor and fragrance compound using Biplot Statistical method in gas chromatography systems. IOP Conf. Series: Mat. Sci. and Eng. 2019 523 012007 doi:10.1088/1757-899X/523/1/012007
CrossRef - Sandra, P. and Bicchi, G. Gas chromatograph mass spectrometry of essential oils. Part 11 capilary Gas analysis. Hue. Ver., He., Bass., 1984 329-357
- McLafferty F. W. Interpretation of Mass Spectra. University Science Books. Maple-vail Book Manufacturing Group, U.S.A. 1993 351-355.
- Kennepohl D. (2022). Athabasca University Chemistry 350 Organic I.available in https://libretext.org Pp281-360
- Compositional Analysis and Antimyco bacterium, Tuberculosis activity of essential oil of Hyptis Spicigera Lamiceae obtained from North-Eastern Nigeria. Int. J. of Sci. and Res, 2015 4(9); 2319-7064
- R.L. Identification of aroma active compounds in orange essential oil using gas chromatography-olfactometry and gas chromatography-mass spectrometry. J. Chro. A. 2003 998 201-211
CrossRef - Adedeji J., Hartman T.G., Rosen R.T. and Ho C.T (1991). Free and glycosidically bound aroma compounds in hog plum (spondias mombins L.) J. of Agri. F. and Chem. 1991 39 1494-1497
CrossRef - M.J., Margaria C.A, Shaw P.E. and Goodner K.L. (2002). Aroma active components in aqueous kiwi fruit essence and kiwi fruit puree by GC-MS and multidimensional GC/GC-O. J. of Ag., F. and Chem. 2002 50 5386-5390
CrossRef - Mohamed A. (2020). β-Caryophyllene: A Single Volatile Component of n-Hexane Extract of Dracaena cinnabari Resin. Mol. 2020 25, 4939; doi:10.3390/molecules25214939
CrossRef - Jirovetz L. Buchbauer G., Stoyanova A.S., Georgiev E.V. and Damianova S.T. (2003). Composition, quality control and antimicrobial activity of the essential oil of long time stored dill (Anethum graveolens L.) seeds from Bulgaria. J. of Ag. F. and Chem. 2003 51 3854-3857
CrossRef
This work is licensed under a Creative Commons Attribution 4.0 International License.