Photocatalytic Degradation of New Fuchsine using Lead Chromate for Water Reuse: Efficiency and Degradation Pathway Contaminants Remediation
Sarita1*, Suraj Sharma1
, Adhidesh S. Kumawat2
and Kumud Intodia1
1Department of Chemistry, Government Meera Girls College, Udaipur–313001, Rajasthan, India.
2Department of Chemical Engineering, NIT Rourkela, Rourkela, Odisha, India.
Corresponding Author E-mail: choudharysarita110@gmail.com
DOI : http://dx.doi.org/10.13005/ojc/400434
Article Received on : 27 Jun 2024
Article Accepted on : 24 Jul 2024
Article Published : 12 Aug 2024
Reviewed by: Dr. Makeswari R
Second Review by: Dr. Walid rezig
Final Approval by: Dr. Ayssar Nahle
This study investigates a novel property of lead chromate that enhances its effectiveness in degrading organic dye pollutants. This property arises from lead chromate's photocatalytic activity under visible light. Lead chromate samples were prepared using direct co-precipitation. Using the cationic dye New Fuchsine as a model molecule, the catalytic performance of these lead chromate compounds was investigated in order to evaluate their green catalytic activity. In order to assess the lead chromate degradation efficiency, the study looked at a number of experimental parameters, including the pH of the dye solution, contact time, lead chromate dosage, and initial concentration of New Fuchsine dye. Additionally, the study discusses potential mechanisms underlying the breakdown of New Fuchsine facilitated by lead chromate under visible light conditions.
KEYWORDS:Degradation; Lead chromate; New fuchsine; photocatalytic; Recyclability; Visible Light
Download this article as:
Copy the following to cite this article: Sarita S, Sharma S, Kumawat A. S, Intodia K. Photocatalytic Degradation of New Fuchsine using Lead Chromate for Water Reuse: Efficiency and Degradation Pathway Contaminants Remediation. Orient J Chem 2024;40(4). |
Copy the following to cite this URL: Sarita S, Sharma S, Kumawat A. S, Intodia K. Photocatalytic Degradation of New Fuchsine using Lead Chromate for Water Reuse: Efficiency and Degradation Pathway Contaminants Remediation. Orient J Chem 2024;40(4). Available from: https://bit.ly/3yy4Df6 |
Introduction
The swift advancement of diverse manufacturing technologies, including textiles, food production, leather, paper, and printing, significantly improved human living standards. The environment and the health of people and animals on Earth can be harmed by organic dyes found in wastewater released by numerous companies, including those that produce food, plastics, and textiles 1. Industries often discharge residual dyes and other pollutants directly into water bodies, causing pollution. Photocatalysis is regarded as one of the most promising methods for breaking down toxic organic pollutants in various industrial effluents 2. For instance, rhodamine B (RhB) can naturally degrade anaerobically to produce aromatic amines that may be carcinogenic. The primary techniques used in traditional wastewater treatment are chemical, biological, and physical. These approaches, however, are unable to provide a comprehensive solution. The concentration of dye in water can be reduced by physical techniques like sorption and membrane filtration, as well as chemical techniques like flocculation in conjunction with flotation and filtration. However, these dyes cannot be broken down into non-polluted compounds. However, biological techniques like anaerobic and aerobic microbial degradation take a long time and don’t work well for many hues 3. Metal oxides, metal sulfides, and oxy halides are common in nature and are employed extensively as photocatalysts on a huge scale. This is because of their ability to produce charge carriers, remarkable longevity throughout several phases, and biocompatibility4.
Remediating water pollution may benefit from the use of the binary compound SrAl2O4:Eu2+, Dy3+/g-C3N4, which effectively degrades basic fuchsine and exhibits enhanced photocatalytic performance, durability, and reusability5. After being effectively synthesized, analyzed, and optimized for basic fuchsine photodegradation, the high-efficiency, recoverable Ag2CrO4/Ag/Fe3O4/RGO photocatalyst (AAFR-NCPs) achieved an 80.15 % degradation efficiency under ideal conditions6.
Kokal et al.7 developed Eco-friendly, inexpensive dye-sensitized solar cells using New Fuchsine dye, an aqueous electrolyte, and counter electrodes that are free of platinum. Roushani et al.8 have explored the use of graphene quantum dots as innovative and eco-friendly nanomaterials for the breakdown of cationic dyes by photocatalysis, including New Fuchsine, under visible light. Gupta et al.9 utilized electrospun, self-assembled ZnO nanofiber structures to achieve photocatalytic degradation of acid fuchsine dye under natural solar radiation.
Kushare et al.10 developed a novel recoverable catalyst, the CoCr2O4@GeO2@ZnO core-shell nanoparticle, which they prepared, characterized, and used for the photocatalytic degradation of basic fuchsine dye.
Hamza and Rahman et al.11 investigated a simple one-pot solid-state method to fabricate a novel binary nanocomposite of commercial ZnO and PbCrO4, which demonstrated enhanced photocatalytic degradation of Rhodamine B dye. Sabzehmeidani et al.12 investigated the sonophotocatalytic treatment of rhodamine B using a visible-light-driven composite of CeO2/Ag2CrO4 in batch mode. The CeO2 nanofibers, synthesized via electrospinning into ribbon-like structures, were a key component in their study. Abbasi et al.13 conducted a straightforward synthesis of PbCrO4 and PbCrO4/Ag nanostructures, demonstrating their effectiveness as photocatalysts for degrading organic contaminants. Abou-Gamra, Ahmed, and Hamza et al.14 researched the use of commercial PbCrO4/TiO2 for the photodegradation of rhodamine B in aqueous solutions caused by visible light.
Durmus et al.15 investigated the graphene oxide/zinc oxide (GO/ZnO) nanocomposite’s photocatalytic activity. The composite was synthesized using a two-step sol-gel deposition process and demonstrated effective photocatalytic activity in degrading basic fuchsine (BF) dye. Their findings indicated that this nanocomposite structure reduced the band gap of ZnO nanoparticles, enhancing their photocatalytic efficiency for degrading BF dye in aqueous solutions.
Zhang et al.16 synthesized nano-lead chromate (PbCrO4) via chemical precipitation to address water-soluble Cr (VI) leaching from COPR through simple water washing. They utilized this material for degrading methylene blue (MB).
In international scientific notation, 4-[(4-amino-3-methylphenyl) (4-imino-3-methylcyclohexa-2, 5-dien-1-ylidene) methyl]-2-methylaniline hydrochloride is known as New Fuchsine. The chemical formula for it is C22H24ClN3 and a molar mass of 365.91 g/mol. This compound is soluble in water and appears as an odorless dark green powder. New Fuchsine finds applications in biology and chemistry, notably as a cationic dye in the textile industry and as a redox indicator in analytical chemistry. Limited research has explored PbCrO4 as a photocatalyst; therefore, this study investigates PbCrO4 efficacy in degrading New Fuchsine.
![]() |
Table 1: The composition and properties of the dye |
Experimental Analysis
Materials and Reagents
Lead chromate was created by co-precipitating it with potassium chromate and water-soluble lead nitrate in a wet chemical procedure. Distilled water was added to separate beakers of 27.60 g of lead nitrate and 16.182 g of potassium chromate. When potassium chromate and lead nitrate solutions are combined, precipitates of lead chromate with a deep lemon-yellow hue result. It was then filtered, thoroughly rinsed in water, and baked at 100 °C.
Photocatalytic process
The degradation rate of New Fuchsine dye served as a measure of the catalyst’s photocatalytic performance. To prepare a stock solution of the dye at 1.0×10-3 M concentration, In 250 milliliters of double distilled water, 0.0914 g of the dye was dissolved. Using a digital pH meter (Systronics model 335), the pH of the dye solution was checked, and any necessary corrections were performed with standard solutions of 0.1 N hydrochloric acid or 0.1 N sodium hydroxide. Every ten minutes, 3 mL of the reaction mixture containing 0.10 g of photocatalyst was removed for examination under the light of a 200 W tungsten lamp.
Absorbance (A) at λmax = 546 nm was measured, with thermal radiation blocked by a water filter. The light source and reaction mixture’s distance were changed based on the light intensity, which was measured with a Suryamapi (CEL model SM 201). We measured the absorbance of the solution at different time intervals using a spectrophotometer (Systronics Model 106). The results showed that absorbance decreased with increased exposure time, indicating a reduction in the concentration of New Fuchsine dye over time.
Results and Discussion
The following equation was used to get the rate constant:
K = [2.303 × slope]
Typical Run
Fig. 1 shows a graphic representation of the usual run, with a rate constant of 1.18 × 10-4 s-1. Light intensity = 70.0 mWcm−2, pH = 8, [New Fuchsine] = 1.8×10−5 M, Semiconductor =0.04 g.
![]() |
Figure 1: Typical run |
Effect of Parameters
pH Variation
New Fuchsine’s photocatalytic degradation rate has been found to rise in the pH is raised, achieving its highest point at pH= 8. The reaction’s pace dropped as the pH was raised more. This behavior can be explained by considering that increasing the pH enhances the likelihood of generating oxygen anion radicals (O2•−). These radicals are produced when O2 molecules react with electrons (e–) in the semiconductor’s conduction band. Superoxide anion radicals can react with protons to create HO2• radicals, and the basic range is generally the optimal pH range for these reactions. The pace at which the dye is broken down by photocatalysis rises with the amount of HO2• radicals produced. The rate of New Fuchsine’s photocatalytic breakdown was found to decrease above pH=8. This decrease could be explained by the neutralization of New Fuchsine from its cationic form, which lessens the adsorption of –OH ions and therefore the compound’s attraction to the negatively charged semiconductor surface.
The [New Fuchsine] value is 1.8 × 10−5 M, the semiconductor weighs 0.04 g, and the light intensity is 70 mWcm−2 as shown in Fig.2.
![]() |
Figure 2: pH Variations |
Dye Concentration Variation
Fig.3 presents the findings regarding the impact of dye concentration on the photocatalytic degradation of New Fuchsine, investigated within the range of 1 × 10-5 to 2.4×10-5 M. However, at approximately 1.8 × 10-5 M (the optimal condition), the efficiency of photocatalytic degradation started to decline. As dye concentration was raised, it was noticed that dye degradation increased. In this situation, the dye will begin to function as an internal filter, which will prevent the intended.
![]() |
Figure 3: Concentration of Dye Variation |
Composite Variation
The study explored the effect of varying catalyst amounts (between 0.02 and 0.18 g) on the rate at which dyes deteriorate. Fig. 4 illustrates how the rate constant varies with different semiconductor quantities. It was observed that increasing the amount of photocatalyst enhances the rate of photocatalytic activity. Specifically, a semiconductor dosage of 0.04 g resulted in the highest degradation rate. However, beyond 0.04 g, the rate significantly dropped constant. This decline is likely due to the increased thickness of the photocatalyst layer rather than an increase in exposed surface area, as evidenced by experiments using vessels of different dimensions.
![]() |
Figure 4: Variation of Composite |
This decrease may also be attributed to potential light obstruction caused by excessive amounts of photocatalyst. The experimental conditions were pH = 8.0, [New Fuchsine] = 1.8 × 10-5 M, and 70.0 mWcm-2 is the light intensity.
Light Intensity Variation
To investigate how light intensity affects photocatalytic degradation, distances ranging from 20 to 70 mWcm-2 was retained in the space between the photocatalyst’s exposed surface and the light source. Fig. 5 depicts rate constants at various light intensities. It was shown that New Fuchsine’s photocatalytic degradation depended more on increasing light intensity because more photons will strike a given area of the photocatalyst surface in a given amount of time. The highest degradation rate of New Fuchsine was noted at 70.0 mWcm-2. This could be attributed to thermal effects or secondary reactions. pH = 8 , [New Fuchsine] = 1.8 × 10−5 M, Semiconductor = 0.04 g.
![]() |
Figure 5: Variation of Light Intensity |
Mechanism
On the basis of these findings, the following basic mechanism for the photocatalytic degradation of New Fuchsine dye has been proposed:
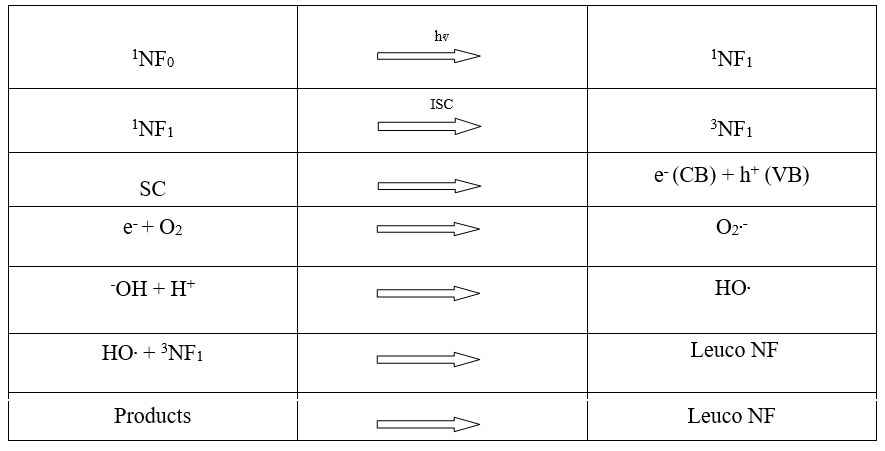
New Fuchsine absorbs radiation at the appropriate wavelength, becoming excited and reaching its first excited singlet state. To get to a more stable triplet state, it also goes through intersystem crossover (ISC). This energy is simultaneously used by the semiconductor lead chromate (SC) to promote an electron from the valence band to the conduction band. Dissolved oxygen in the medium abstracts this electron, forming a superoxide anion radical (O2•). In the basic environment, this anion radical combines with a proton to generate HO2• radicals. HO2• radicals oxidize New Fuchsine may further break down into end products in its leuco state. Surprisingly, the hydroxyl radical scavenger 2-propanol did not considerably alter the rate of New Fuchsine degradation, suggesting that the OH radical is not actively involved as an oxidizing species in this process.
Scavenger test
In this study, several scavengers, such as p-benzoquinone (BQ) for •O2−, isopropyl alcohol (IPA) for •OH, and potassium iodide (KI) were employed. Fig. 6 clearly shows that all scavengers contributed to reducing the degradation of New Fuchsine dye to some extent. Specifically, the degradation of New Fuchsine decreased by 25 %, 8 %, and 22 % in the presence of p-benzoquinone, KI, and IPA, respectively. These findings from different quenchers suggest that •OH contributes significantly to the New Fuchsine’s photocatalytic breakdown.
![]() |
Figure 6: Scavenger test with degradation |
Stability and reusability of Lead Chromate
Four consecutive photocatalytic experimental runs using recycled Lead Chromate photocatalyst and New Fuchsine solutions were conducted to assess the reusability of Lead Chromate. Up to four rounds of the New Fuchsine’s breakdown by Lead Chromate particles’ activity were noted. By employing the same batch of lead chromate catalyst in subsequent tests to degrade new fuchsine, PbCrO4‘s stability and reusability are assessed. According to Fig. 7, the New Fuchsine photodegradation efficiency was 64 % on the first run and 48 % on the fourth run. The minor drop in effectiveness indicated that synthetic PbCrO4 would be a good catalyst for use in real-world applications.
![]() |
Figure 7: Stability and Reusability |
Conclusion
Lead Chromate was synthesized and evaluated for its ability to photocatalytically degrade New Fuchsine dye. The study demonstrated that New Fuchsine dye could be effectively degraded using Lead Chromate as a photocatalyst under visible light with optimized conditions. Furthermore, the prepared Lead Chromate could be reused up to four times without experiencing a notable decline in its photocatalytic activity as shown in Fig.8.
![]() |
Figure 8: Practical Set up |
Acknowledgment
We extend our sincere gratitude to the faculty of the Department of Chemistry at Government Meera Girls College, Udaipur, for their invaluable cooperation in this research endeavor.
Conflicts of Interest
No conflicts of interest are disclosed by the authors.
References
- Regan, H. Asian Rivers are turning black. And our colorful closets are to blame. CNN Style, 2020, 28.
- Wei, T., Gao, S., Wang, Q., Xu, H., Wang, Z., Huang, B., & Dai, Y. Transformation from Ag@ Ag3PO4 to Ag@ Ag2SO4 hybrid at room temperature: preparation and its visible light photocatalytic activity. Journal of Nanoparticle Research, 2017, 19, 1-13.
CrossRef - Natarajan, S., Bajaj, H. C., & Tayade, R. J. Recent advances based on the synergetic effect of adsorption for removal of dyes from wastewater using photocatalytic processes. Journal of Environmental Sciences, 2018, 65, 201-222.
CrossRef - Khan, M. M., Adil, S. F., & Al-Mayouf, A. Metal oxides as photocatalysts. Journal of Saudi chemical society, 2015, 19(5), 462-464.
CrossRef - Li, S. S., Liu, M., Wen, L., Xu, Z., Cheng, Y. H., & Chen, M. L. Exploration of long afterglow luminescent materials composited with graphitized carbon nitride for photocatalytic degradation of basic fuchsin. Environmental Science and Pollution Research, 2023, 30(1), 322-336.
CrossRef - Shariati, M., Babaei, A., & Azizi, A. Synthesis of the tetranary magnetic nanocomposite as a good photocatalyst for degradation of basic fuchsin dye in aqueous media under visible light: Characterization, response surface methodology, and kinetic study. Journal of Materials Research, 2023, 38(10), 2666-2678.
CrossRef - Kokal, R. K., Bhattacharya, S., Cardoso, L. S., Miranda, P. B., Soma, V. R., Chetti, P., & Raavi, S. S. K. Low cost ‘green’dye sensitized solar cells based on New Fuchsin dye with aqueous electrolyte and platinum-free counter electrodes. Solar Energy, 2019, 188, 913-923.
CrossRef - Roushani, M., Mavaei, M., Rajabi, H.R. J. Molecu. Catal. A: Chemical, 2015, 409, 102-109.
CrossRef - Gupta, A., V Nandanwar, D., R Dhakate, S. Advan. Materi. Lett. 2015, 6(8), 706-710.
CrossRef - Kushare, S.S., Bobade, V.D., Tope, D.R., Borhade, A.V. J. Ind. Chemi. Soc., 2021, 98(12), 100239.
CrossRef - Hamza, M.A., Abd El-Rahman, S.A., Abou-Gamra, Z.M. Opti. Mater. 2022, 124, 111987.
CrossRef - Sabzeh Meidani, M.M., Karimi, H., Ghaedi, M. Enviro. Sci. Pollu. Res., 2019, 26, 8050-8068.
CrossRef - Abbasi, A., Hamadanian, M., Gholami, T., Salavati-Niasari, M., Sadri, N. Separa. Purifica. Techno. 2019, 209, 79-87.
CrossRef - Abou-Gamra, Z.M., Ahmed, M.A. Hamza, M.A. Nanotechnol. Environ. 2017, Eng. 2, 12. https://doi.org/10.1007/s41204-017-0024-9.
CrossRef - Durmus, Z., Kurt, B. Z., & Durmus, A. 2019, Synthesis and characterization of graphene oxide/zinc oxide (GO/ZnO) nanocomposite and its utilization for photocatalytic degradation of basic fuchsin dye. ChemistrySelect, 4(1), 271-278.
CrossRef - Zhang, D., Zhang, X., Zhang, Z., Zhang, X., Qiu, F., Liu, Z., & Li, W. J. Clea. Product. 2022, 379, 134352.
CrossRef
This work is licensed under a Creative Commons Attribution 4.0 International License.