Medicinal Utility of Some Schiff Bases and their Complexes with First Transition Series Metals: A Review
Tahmeena Khan1*, Saima Zehra2
, Almas Alvi2
, Umama Fatima2
and Alfred J. Lawrence2
1Department of Chemistry, Integral University, Lucknow-226026, India.
2Department of Chemistry, Isabella Thoburn College, Lucknow-226007, India.
Corresponding Author E-mail: tahminakhan30@yahoo.com
DOI : http://dx.doi.org/10.13005/ojc/370506
Article Received on : 31-Aug-2021
Article Accepted on :
Article Published : 27 Oct 2021
Reviewed by: Dr. Tasneem Mohammed

Second Review by: Dr. Lokendra Singh
Final Approval by: Dr. Nenad Ignjatovic
Schiff based ligands and their complexes have emerged as potential drug candidates. Owing to their excellent chelating tendency, they easily coordinate with transition metals which have vacant orbitals. Transition metal complexes have several advantages because of their better acceptability and low toxicity in biological systems. These metals also serve as micronutrients and as co-factors of various metallo-enzymes which justifies the need of their designing and synthesis. Many modifications have been suggested in the ligand moiety for the purpose of activity enhancement and some of them have been described in the present review. These modifications have enhanced better potency against a number of diseases and resulting in low toxicity and better solubility in vivo. The transition metal complexes with Schiff based complexes have exhibited an array of activities including anticancer, antioxidant and antimicrobial. Their analytical applications have also been reported. The present review summarizes some of the recent advances in the field of synthesis and designing of new Schiff based complexes particularly with first transition series metals and their medicinal applications.
KEYWORDS:Coordination; Medicinal; Schiff bases; Transition Metals
Download this article as:
Copy the following to cite this article: Khan T, Zehra S, Alvi A, Fatima U, Lawrence A. I. Medicinal Utility of Some Schiff Bases and their Complexes with First Transition Series Metals: A Review. Orient J Chem 2021;37(5). |
Copy the following to cite this URL: Khan T, Zehra S, Alvi A, Fatima U, Lawrence A. I. Medicinal Utility of Some Schiff Bases and their Complexes with First Transition Series Metals: A Review. Orient J Chem 2021;37(5). Available from: https://bit.ly/3vOuVUm |
Introduction
Cations are positively charged ions and are important for maintaining charge balance. Transition metal cations are known to neutralize charges on the residues of proteins, DNA and RNA 1. Transition metals, also known as d block elements belong to groups 3-12 in the long form of periodic table. They have vacant d orbitals in their penultimate (n-1) shells and placed between the s and p blocks, hence, they are commonly referred to as transition elements. They usually exhibit variation in their oxidation states making them suitable for coordination complexes. Although transition metal ions are lesser common in nature, yet they play a critical role in structural and catalytic chemistry 2. Iron (Fe) is an important metal and having crucial role play in the functioning of the respiratory proteins and other proteins involved in electron transport 3. The metal exists in +2 and +3 oxidation states, and has structural and catalytic roles. Like Fe, other first series transition elements have medicinal applications and are found as crucial components of several drugs, pharmaceutical and diagnostic agents. Several other first-series transition metals like copper, zinc, manganese and cobalt are considered as essential elements and required for life and their absence will lead to the death of organism in severe cases. Nickel (Ni), another element of first transition series is essential for the activity of urease, a bacterial enzyme needed for its growth but it is not essential for human beings. Depending upon the ligand and redox environment, the first-series transition elements exhibit, several oxidation states 4. +2 and +3 being the most common oxidation states, vanadium also exists in IV and V oxidation states. Ti, V, Cu, and Zn form stable four-coordinated complexes, whereas most of the first-series transition metals form six-coordinated complexes [5]. The metal ions in low oxidation state prefer to coordinate with N containg ligands, whereas metal cations in higher oxidation states tend to coordinate with O containing ligands. The functioning of metal-based drugs is different from carbon-based drugs because the carbon-based drugs breakdown into CO2 and a number of metabolites during the metabolism, whereas the metal based drugs upon metabolism produce metal ions which interact with different cellular components leading to the formation of new compounds having biological consequences.
Metal-ligand chemistry
Transition metals like Mn, Co, Zn, etc. are found to be essential for life. Few of the metals like Co, Mn, Zn have therapeutic importance associated with them. Their in vivo medicinal utility is also influenced upon coordination with different ligands how the solubility of the complex is altered which also affects their distribution. Effective distribution of metal ions in the body also depends upon the concentration of low molecular weight ligands or protein complexation sites 7. There are a variety of biological ligands available in the biological fluids to bind with the metal ions which have a number of complexing sites available for binding like in the case with Fe and Cu in low molecular weight peptides. The activity of the metal in complexed form may be dependent on its concentration. For instance, in the Pt based anticancer drugs, the required quantity is in milligram range. Platinum binds with DNA and may also interact with cellular and extracellular fractions. The binding of the metals ions with appropriate ligands is largely governed by the Hard Soft Acid Base (HSAB) Concept. According to the concept, metal ions with high positive charge and small size are considered as hard and those with low positive charge density like Na+. K+, Cr3+ and cations like Pt2+, Cu+, Cd2+ etc. which are bigger in size are projected as soft. The dipositive metal ions of the first transition series lie between these two categories and have an affinity for ligands with O, N, S and P donor atoms containing ligands. Consequently, Cu(I) is more capable to complex with caeruloplasmin and with S containing ligands. Transition metals indulge in coordinate covalent bonding resulting in fixed directional bonds. So, the properties are dependent on the nature of the ligand as well as the metal ion. Usually tetrahedral and octahedral geometries are acquired by transition metals and irregular geometries are attained when a solitary electron is present in d orbitals like Cu(II) with d9 configuration prefers to attain tetragonal geometry and Zn(II) with d10 configuration prefers tetrahedral structure. The size of the metal ion also affects the reactivity of the complex [7]. Redox potential and catalytic properties are also influenced by structural alterations like steric hindrance caused by the ligand or the electronic structure. The stability of the complex depends upon the thermodynamic and kinetic factors. Co3+, Cr3+, Ni2+ react slowly as depenedent on the kinetic factors, whereas the bonding interactions are related to the thermodynamic factors.
Transition metals and therapeutic importance
Alfred Werner proposed his coordination theory in 1870’s based on compounds, containing cobalt chloride and ammonia. For his breakthrough studies he was awarded the Noble prize in 1913. Cisplatin or cis-diammine-dichloridoplatinum (II) was discovered in 1960 for its anti-tumor action and for the treatment of solid carcinomas [8]. The mechanistic action of cisplatin involved movement in the cell via diffusion and active transport. The compound caused platination of DNA involving cross-linkage of inter and intra strands involving formation of addition products, usually with guanine residue because of its electron richness. Formation of adducts with cisplatin caused distortion and inhibition of DNA synthesis 9. These DNA adducts can also bind to cellular proteins like repair enzymes, histones etc. 10 . And also to HMG-domain protein, thereby enhancing the anticancer potential. However, cisplatin was also found to bind with negatively charged species like metallothioneins and glutathiones which are needed to confiscate heavy metals and eliminate them from the cell. Drug resistance was another major issue faced by cisplatin in cancer chemotherapy. Cisplain also met with several harmful side effects, as it affected the rapidly dividing normal tissues like hair follicle, bone marrow and the cells of gastrointestinal tract. Trans DDP had an altogether different binding mode with the cellular DNA and found to act on cisplatin resistant tumors. After the discovery of cisplation many other transition metal complexes were studied for their medicinal potential. Titanocene; an organometallic compound containing Ti has been effective against breast cancer and gastrointestinal cancer cells 7. Pt and Pd complexes in +2 oxidation state are photo-sensetive and cause, cross linking with HMG protein, thereby inhibiting RNA transcription 11. Gold complexes have been shown to target mitochondrial site in cancer cells. Au complexes containing bipyridyl ligand exhibited anticancer activity 12. The 2-[(dimethylamino) methyl] phenyl gold (III) complex has exerted anti-cancer potential against various human cancer cell lines 13. In another instance, gold particles used in conjugation with radio and chemotherapy have found to enhance DNA damage and been more target specific 14. First transition series metal complexs like Mn (III) complexes have found to prompt apoptosis. Fe complexes have shown potential anticancer activities 15, for instance ferrocifenes (Fig. 1) have demonstrated anticancer activity against breast cancer cells 16 and its derivatives containing hydroxyl groups have been found to have a high affinity for estrogen receptor. Copper (I) compounds have been shown to produce reactive oxygen species (ROS) in their anticancer activity. Cu(I) reduces H2O2 to OH–. Superoxide anion or glutathione reduces Cu(II) to Cu(I), therefore the production of ROS like OH. is facilitated by Cu, irrespective of the initial form.
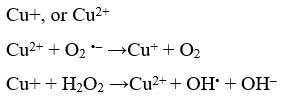
![]() |
Figure 1: Ferrocifen. |
uperoxide anion (O2•-) results from the reduction of the molecular oxygen taking place in several biological processes changes into H2O2 via dismutation 17. [Cu (thp)4][PF6] (Fig. 2) has exhibited 40 times higher cytotoxicity than cisplatin in colon cancer cells. Cu(I) complexes possessing heteronuclear bases like pyridine, bipyridine, phenanthroline etc. have also exerted anticancer effect on numerous cell lines displaying that coordination of more than one type of ligands can enhance the activity noticeably. Fe, Cu, Co, Mn, etc. may undergo redox reactions due to the variability in oxidation states resulting in DNA strand breakage. The mechanistic action of bleomycin and anticancer antibiotic also involves chopping of DNA strand facilitated by Fe binding of the drug 18. Ru complexes in +2 and 3 oxidation states have been effective in human ovarian cancers and metastasis cancers. Ruthenocene derivatives also behave as antiestrogen 19. Ruthenocene complexes have better binding potential than hydroxyl tamoxifen, a prospective antagonist for estrogen. Many of Ru complexes have shown anti-estrogen properties similar to Tamoxifen 20-21. Two Ru complexes are under clinical trial to be qualified as anticancer drugs- NAMI-A and KP1019. KP1019 is active against primary cancers whereas NAMI-A works against secondary metastatic cancer cells 22. La has been found to treat various cancers. Other than anticancer activity transition metals have been used as effective antimicrobial agents also. Ag and Hg have been long used as antimicrobial agents 23. Ag is less toxic comparing with other transition metals. Silver (I) sulfazine is used to cure serious burns and to avert bacterial infection 2. It has a polymeric structure facilitating slow release of antibacterial Ag+ ion. Chlorohexidine- Silver Sulfadiazine is used in catheter infection as well 24. The metal compounds act by measured discharge of the active metal ion. Inhibition of thiol function occurs in bacterial cell wall giving a reasoning of bacteriocidal action. Organometallic compounds of several transition metals have been shown to have trypanocidal activity 25.
![]() |
Figure 2: [Cu (thp)4][PF6 |
Zn compounds have been used to treat diarrhea. Zn has also been used for regulation of hemodialysis patients. Mn and its compounds have been tested against various microorganisms 26. The mechanistic action of the transition metal complexes is due to the fact that the ligands share their electron pairs with the metal atoms leading to the delocalization of electrons over the chelate. Chelation promotes the permeation in cell membrane. In a nutshell the utility of transition metal complexes is because of the variability in oxidation states, and different coordination numbers leading to different geometries. The ligand to which the metal ion binds regulates the activity of the metal and plays an important role in the establishment of secondary coordination sphere which is involved in the identification of biological targets as DNA, enzymes and protein receptors. The ligands also take part in significant biological redox reactions, like in vivo hydrolysis meditated by metals. These factors can lead to potential diversity in the designing of metal based drug compounds.
Schiff based ligands
Schiff based ligands have gained considerable importance as their metal chelates act as potent anticancer, antibiotic, anti tuberculosis, antipyretic agent as well as anti fertility agents. Schiff’s bases have potential industrial applications also, including catalytic properties, in perfumery, as fibers, plant growth inhibitors, oxygen absorbents, polymers, lubricating agents as removal agents for metallic impurities and as drying agents 27-28. They are generally prepared as condensation products of primary amines with carbonyl compound and named after their discoverer Hugo Schiff in 1964 generally containing an azomethine group (RHC=N-R’) where R and R’ represent alkyl, aryl, cyclo alkyl or heterocyclic moieties or their substitutes (Fig. 3). A large number of transition metal complexes are known to possess utility in medicinal chemistry 29-30. The azomethine nitrogen is sp2 hybradized with a lone pair of electrons available for donation. The chelation tendency further increases if the N is present near to any electron donating group. Few examples of Schiff bases are given as Fig.(s). 4-6.
![]() |
Figure 3: General structure of Schiff’s base |
![]() |
Figure 4: Few Examples of Bidentate Schiff bases |
![]() |
Figure 5: Few Examples of Tridentate Schiff bases |
![]() |
Figure 6: Few Examples of Tetradentate Schiff bases |
Synthesis of Schiff’s bases
Schiff’s bases can be prepared through a number of reaction pathways. The most common pathway includes condensation between a suitable amine and an aldehyde or ketone under refluxing conditions in acidic conditions (Fig. 7). The reaction involves the attack of electron rich nitrogen on the carbonyl carbon, leading to an unstable carbiolamine intermediate (Fig. 8). After the removal of hydroxyl group, C=N is formed, which is known as imine. The reaction depends on several factors including the pH of the solution and steric and electronic effects of the reacting carbonyl compound and amine. In acidic medium protonation of amine takes place. However, only a small amount of acid is required, otherwise carbinolamine would not be formed. On the contrary in very basic condition, the reaction is hindered due to the unavailability of necessary protons to facilitate the removal of the carbinolamine hydroxyl group. In comparison to ketones, aldehydes are faster to react, in the condensation reaction as the reaction centre in aldehydes is less sterically hindered. Furthermore, the carbon atom in ketones donates electrons making it less electrophilic in nature as compared to aldehydes 31. The rate determining step of the reaction is when dehydration takes place. Removal of water is necessary for product formation as acids or bases may hydrolyze Schiff bases. Water in the reaction is removed from molecular sieves 32 or by dehydrating solvents like tetramethyl orthosilicate or trimethyl orthoformate 10. Lewis acids and Bases like ZnCl2, TiCl4, H2SO4, NaHCO3, MgSO4, Mg(ClO4)2, Er(OTf)3 are used in the synthesis of Schiff bases 33.Solvent free, microwave, IR radiation, etc. have also been found to assist the reaction. Microwave irradiation finds particular application due to the simplicity of operation and non-involvement of organic solvents. In actuality the formation of Schiff bases include elimination after addition. Purification of Schiff bases is also not advisable because they may undergo hydrolysis on silica gel.
![]() |
Figure 7: General synthesis reaction of Schiff base |
![]() |
Figure 8: Mechanism of action of general synthesis reaction of Schiff base |
Specific applications of Schiff bases
Antibacterial activity
Drug resistance is a major obstacle linked to increased mortality rate associated with infectious diseases. Therefore new drugs with more effective mechanisms are required to overcome the resistance issue. Schiff base N (salicylidene)-2-hydroxyaniline is found to be effective against mycobacterium tuberculosis H37Rv 34. Schiff bases derived from 5-chloro-salicaydehyde have been found to be effective against Pseudomonas fluorescence with the MIC values between 2.5-5.2 µg/mL 35. Schiff bases derived from isatin have also shown to possess antibacterial activity 36 against E.coli, NCTC 10418, Vibrio cholera, Proteus shigelloides etc. and those derived from isoniazid has been found to be active aginst M. tuberculosis H37Rv, having greater activity than the intermediate formed 37 and found to be non-toxic against VERO cell line, showing that the Schiff base compounds can be used as effective antitubercular drugs.Schiff bases derived from 1.2-benzisothiazole and their cyclic and acyclic derivatives have exhibited antibacterial activity against Gram positive bacteria 38. Antibacterial activity of hydrazide hydrazones and 1.3.4-oxadiazolines of 4-flurobenzoic and hydrazide has been evaluated against S. aureus and E. coli with comparable activity as ceftriaxone 39. The in vitro antibacterial activity of Schiff bases derived from 2- hydroxy-1-naphthaldehyde with glycine, L-alanine L-phenylalanine, L-histidine, L-tryptophane was tested and it was found that the antimicrobial activity decreased with the increasing size of the amino acid residue 40.
Antiviral activity
Viral diseases may be life threatening especially for those with low or suppressed immunity. The antiviral drugs available are not completely effective and may involve a number of side effects. Mutation of virus is a major hurdle for the development of therapeutic interventions as antiviral agents. Schiff bases derived from salicaldehyde and 1-amino-3-hydroxy-guanidine tosylate are found to be effective as antiviral agents 41. 1-amino-3-hydroxyguanidine tosylate-derived Schiff bases, 2- (3-allyl-2-hydroxybenzylidene)-N-hydroxy hydrazine carboximidamide derivative has been effective against mouse hepatitis virus (MHV). Schiff bases derived from 3-(benzylideneamino)-2- phenylquinazoline-4(3H)-ones were prepared through substitution with different carbonyl compounds. Their antiviral activity was evaluated against herpes simplex virus-1 (KOS), herpes virus-2 (G), vesicular stomatitis virus, herpes simplex virus-1 TK-KOS ACVr, para influenza-3 virus, reovirus-1 42. Abacavir derived Schiff bases have been synthesized and assessed for their antiviral activity as abacavir is capable of inhibiting the activity of reverse transcriptase 43. The Schiff bases showed activity against HIV-1.
Anti-malarial activity
Malaria is often neglected as a disease although annually 500 million people are affected by the disease. P. falciparum, P. vivax, P. ovale, and P. malariae are mainly responsible for human malaria. Schiff base derived from 5-nitroisoquinolines has been tested against ACC Niger chloroquine resistant P. falciparum. N-[(1E)-(5- nitro-1-naphthyl)methylene]-1-[2-(trifluoromethyl)phenyl]methanamine was found to be an effective antimalarial agent with an IC 50 concentration of 0.7 µg/mL 44. Ancistrocladidine possessing an imine group has been used as a scaffold for its activity against P. falciparum K1 and 3D7 with MIC of 0.3 and 1.9 µg/mL 45.
Antifungal activity
Systematic fungal infection risk is a matter of concern for people with suppressed immunity like those suffering from AIDS, or undergoing cancer therapy and organ transplantation. N-(Salicylidene)-2-hydroxyaniline has been shown to suppress Alternaria brassicae and Alternaria brassicicola, which are phytopathogenic fungi at 500 ppm concentration 46. Chitosan derived Schiff bases have suppressed the growth of Botrytis cinerea and Colletotrichum lagenarium at 1000 ppm 47. 2,4-dichloro-5-flurophenyl Schiff bases have found to inhibit the growth of Aspergillus fumigates, and Aspergillus flavus etc. between 6.3-12.5 µg/mL dose range 48. Schiff bases derived from piperonyl have inhibited the growth of Trichophyton rubrum and E. floccosum 49. The isatin derived Schiff bases have been found to be active against M. audouinii and M. gypseum 50.
Anti-inflammatory activity
Schiff bases derived from dipropylenetriamine and 2-thiophene-carboxaldehyde have been tested for their anti-inflammatory activity 51. Schiff bases derived from 3-(4-(benzylideneamino) phenylimino) 4-fluoroindolin-2-one have been tested for their anti- inflammatory properties and found to possess significant activity comparable to diclofenac 52. 4-aminoantipyrine (4-amino- 1,5-dimethyl-2-phenylpyrazole-3-one) and benzaldehyde derivative has been tested as a new therapeutic agent against oxidative stress and anti-inflammatory agent 53. Schiff bases of 4-aminophenazone have been screened for their anti-inflammatory activities using the Carrageen an-induced paw oedema (CIPO) and histamine induced paw oedema (HIPO) methods with appreciable inhibition 54.
Anticancer activity of Schiff bases
Cancer continues to be a lethal disease and the treatment of the disease includes surgery and chemotherapy with adverse side effects. Schiff bases have been found to exert appreciable anticancer activity. Schiff bases derived from salicaldehyde L-phenylalanine were tested against K562 tumour cell 55 and those derived from 2-acetylpyridine and L-tryptophans were tested against MDA-MB-231 and found to be potential proteaseome inhibitors 56. Schiff bases derived from reduced amino acids have been evaluated for their DNA interaction with salmon sperm DNA and their anticancer activity has been tested against HL-60, KB, BGC-823 and Bel-7402 cell lines with effective cytotoxicity 57. Schiff bases obtained from S-benzyldithiocarbazate with pyridine-2-carboxaldehyde and salicaldedyhe having ONS and NNS donor atoms have been found to be moderately active against Leukemia 58.
Antidepressant activity
Schiff bases derived from isonicotinoyl hydrazone, N’-[(1Z)-(substituted aromatic) methylidene]pyridine- 4-carbohydrazides synthesized through green chemistry have been evaluated for their antidepressant activity along with their nitro, halo and dimethoxy substituted derivatives which were found to possess significant activity 59.
Anticonvulsant Activity
Anticonvulsant activity of Schiff bases derived from phthalimide, 4-(1,3-dioxo-1,3-dihydro-2H-isoindol-2-yl)- N’-(substituted phenyl) methylene/ethylidene benzohydrazide have been evaluated 60. The compounds were less toxic than the standard compound phenytoin. Through maximal electroshcok seizure (MES) method the anticonvulsant activity of a series of Schiff bases 3,3’{[6-(2,3-dichlorophenyl)-1,2,4- triazine-3,5-diyl]dinitrolo}bis-(substituted-1,3-dihydro-2H-indol- 2-one) was tested and the findings revealed better activity than lamotrigine used as a standard 61. Schiff bases derived from 3-aryl-4(3H)-quinazolinones-2-carboxaldehydes and their thiosemicarbazones have exhibited anticonvulsant activity which may be due to the presence of thiosemicarbazone at the side chain 62.
First Transition series metal complexes with Sciff bases and their medicinal activity
Due to their bi and tri dentate nature Schiff bases form stable complexes with first transition series elements. Derived from 4-amino-5-sulfanyl-1.2.4-tria-zoles and glyoxal, biacetyl or benzyl, complexes of Co and Ni have been prepared in +2 oxidation state and tested for their antibacterial and antifungal activities. Complexes of ONS and NNS containing schiff bases of S-benzyldithiocarbazate (NH2NHCSSCH2Ph) (SBDTC) with pyridine-2-carboxaldehyde and salicylaldehyde, have been synthesized with Ni, Zn, Co, Cu in +2 oxidation state and chromium in +3 oxidation state. The ONS Schiff base possessed moderate activity against leukemia, while its Zn and Co complexes showed significant activity against leukemic cells withDC50 value ranging between 0.35-5.00 63. Schiff based mononuclear complexes with 4-substituted thiazoles and cyclobutane rings, 4-(1- methyl-1-phenylcyclobutane-3-yl)-2-(2-hydroxy-3- methoxy benzylidene hydrazino)thiazole (L1H),methyl-1-p-xylylcyclobutane-3-yl)-2-(2-hydroxy-methoxybenzylidenehydrazino)thiazole (L2H) with transition metal salts of first transition series in +2 oxidation state in EtOH have been evaluated for their antimicrobial activity. Potassium salt of o-hydroxyacetophenone glycine [KHL] and bis(benzylidene) ethylenediamine or thiophene-o-carboxaldene-p-toluidine have been used to synthesize mixed ligand complexes with Cu, Ni, Mn, Co, and Zn salts in +2 oxidation state showing higher antifungal activity than the ligand only 64. Schiff base ligands form complexes easily with Co and have been used in a variety of medical applications. For example, the Co(III) Schiff base compound CTC-96 has completed Phase II clinical trial for the treatment of herpes simplex virus 65. Compound CTC-96 is believed to prevent membrane fusion of the virus. Anticancer activity has been observed in a number of Co(II) and Co(III) Schiff base complexes 66. Salicaldehyde anthranilic acid (SAA) and hetero bases like 2,2’-bipyridine and 1,10-phenanthroline based complexes with oxovanadium have been found to inhibit human protein tyrosine phosphatase, T-cell protein tyrosine and Src homology phosphatase 1 in vivo. The kinetic assay has suggested that the complexes inhibited PTPIB in a reversible manner, indicating their potential as new antidiabitic agents 67. Thiadizole derivatives with salicaldehyde or o-vanillin and their Mo(IV) complex has shown insecticidal properties against Bollworm and promotes cell survival in mung bean sprouts 68. Many Schiff bases have also shown anti- inflammatory, anti-allergy, radical scavenging, analgesic and anti-oxidative properties. Schiff bases derived from thiazole have possessed anti-inflammatory and analgesic properties 69. Anti-tumor activity has been found to increase upon complexation with metals. Cu, Ni, Zn and Co containing complexes with salicaldehyde, 2,4 dihydroxy-benzaldehyde, glycine and L-alanine possessed antitumor activity 70. Schiff bases also abolish enzymatic action in proteins. They have been found to bond with pyridoxal 5’ phoshphate from lysine to alanine or histidine and inhibited the enzymatic activity. Complexes derived from hydrazine carboxomide and hydrazine with Mn(II) have been found to affect reproductive physiology 71. Derived from 2-acetyl pyridine and L-tryptohan (C18H3N3O2) transition metal complexes viz. Cu(C18H16N3O2)2.2CH3OH (1), Zn(C18H16N3O2)2.2CH3CH2OH (2) have been tested for their anticancer potential against MDA-MB-231 breast cancer cell line. The complexes were found to inhibit cellular proliferation 72. Schiff based complexes of Co, Ni, Cu and Zn in +2 oxidation state incorporating indole-3-carboxaldehyde and m-aminobenzoic acid were tested for their antimicrobial activity. Metal complexes have polar and nonpolar properties making them suitable to permeate in the tissues. Chelate formation may increase or decrease the bioactive properties of various organic species 73-74. They can be used to design new, safer therapeutic drugs, yet there is much to explore with respect to the biological potential of the previously known complexes and the yet to be synthesized complexes because of their relatively easy synthetic accessibility by a simple one pot condensation of a carbonyl compound with primary amines. Some more Schiff bases are given in Table 1 and different bonding modes with transition metals are represented in Fig(s). 9-11.
![]() |
Figure 9: Few transition metal complexes with bidentate Schiff bases |
![]() |
Figure 10: Few transition metal complexes with tridentate Schiff bases |
![]() |
Figure 11: Transition metal complex with tetradentate Schiff base |
Table 1: Examples of Schiff bases with their biological activity tested
S.No. |
SCHIFF BASE |
METAL |
ACTIVITY TESTED |
REFERENCE |
1. |
N, N’bis(pyrrole-2-carbaldehyde)ethylenediamine |
Co(II),Ni(II),Cu(II) |
Antimicrobial |
[75] |
2. |
2N-salicylidene-5-(p-nitro phenyl)-1,3,4-thiadiazole |
Co(II) |
Antibacterial |
[76] |
3. |
N,N’-bis(1-(1H-pyrrol-2-yl)ethylidene)propane-1,3-diamine |
Fe(II),Co(II), Ni(II),Cu(II),Zn(II) |
Antibacterial |
[77] |
4. |
Bis[N-(p-tolyl)imino]acenaphthene |
Cu(II) |
Antitumor |
[78] |
5. |
5-dimethyl-2-phenyl-4-[(pyridine-2-ylmethylene)-amino]-1,2-dihydro-pyrazole-3-one |
Cu (I)
|
Anticancer |
[78] |
6. |
2-[{[2-(dimethylamino)ethyl]imino}methyl]6-methoxyphenol |
Co(II) |
Anticancer |
[78] |
7. |
(2-(5-iodo-2-methyl-1H-imidazol-1-yl)-N′-((pyridin-2-yl) methylene)acetohydrazide) |
Cu(II), Co(II), Ni(II) |
Antifungal |
[79] |
8. |
2-(((3-mercapto-5-(pyridin-4-yl)-4H-1,2,4-triazol-4-yl)imino)methyl)-4- methoxyphenol |
Co(II), Ni(II), Cu(II), Zn(II) |
Anticancer |
[80] |
9. |
2-(((3-mercapto-5-(pyridin-3-yl)-4H-1,2,4-triazol-4-yl)imino)methyl)-4- methoxyphenol |
Co(II), Ni(II), Cu(II), Zn(II) |
Anticancer |
[80] |
10. |
N,N’-propane-1,3-diylbis[1-(pyrrol-2- yl)methanimine] |
Co(III) |
Antibacterial |
[81] |
11. |
1-[(thiophen-2-ylmethylidene)amino]propan-2-amine |
Co(III) |
Antibacterial |
[81] |
12. |
1-[(3-methylthiophen-2-ylmethylidene)amino]propan-2-amine |
Co(III) |
Antibacterial |
[81] |
13. |
4-(((5-mercapto-4H-1,2,4-triazol-3-yl)imio)methyl)-2-methoxyphenol |
Co(II), Cu(II), Mn(II), Zn(II) and Ni(II) |
Antibacterial |
[82] |
14. |
8-chloro-N′-(4-(dimethylamino) benzylidene)-[1,2,4]triazolo[4,3-a]pyridine-3-carbohydrazide |
V(III),V(II) |
Antifungal |
[83] |
15. |
2-aminomethylthiophenyl-4-bromosalicylaldehyde |
Ni(II), Cu(II), Zn(II) |
Antimicrobial |
[83] |
16. |
Isatin monohydrazone with 2-hydroxynapthaldehyde/substituted salicylaldehyde and heterocyclic nitrogen base 8-hydroxyquinoline |
Co(II), Ni(II), Cu(II) and Zn(II) |
Antibacterial |
[84] |
17. |
3-((2-hydroxy3-methoxybenzylidene)amino)-2-methylquinazolin-4(3H)-one |
Ni(II) |
Antibacterial |
[83] |
18. |
2-((4-(dimethylamino)benzylidene)amino)phenol |
Co(II), Ni(II), Cu(II), Zn(II), Cr(III) |
Antifungal |
[83] |
19. |
2-((3,4-dimethylisoxazol-5-ylimino)methyl)phenol |
Cu(II) |
Antibacterial Antitumor |
[83] |
20. |
2N-salicylidene-5-(p-nitrophenyl)-1,3,4- thiadiazole |
Co(II) |
Antibacterial |
[83] |
21. |
4,4′-bis-({2-[(2-hydroxy-phenylimino)-methyl]-benzylidene}-amino)-biphenyl-3,3′-diol |
Cu(II), Ni(II) |
Antioxident |
[83] |
22. |
N-salicylidene-βalanin |
Zn(II), Cu(II) |
Antioxidant Antidiabetic |
[83] |
23. |
5-chloro-N′-(2-hydroxy-4-phenyldiazenyl)benzylidene-3-phenyl-1H-indole-2-carbohydrazide |
Cu(II), Co(II), Ni(II), Zn(II) |
Antioxidant, Antibacterial, Antifungal |
[83] |
24. |
2-(4-(diethylamino)-2-hydroxybenzylidene)-N-(4-phenylthiazol-2-yl) hydrazinecarboxamide |
Cu(II), Co(II), Ni(II), Zn(II) |
Scavenging, Antioxidant Antimicrobial |
[83] |
25. |
3-(-(3-(-3,5-dichloro-2-hydroxybenzylideneamino) propylimino)methyl)quinoxalin-2(1H)-one |
Co(II), Ni(II), Cu(II), Zn(II) |
Antimicrobial |
[83] |
26. |
2,4-dihydroxybenzaldehyde (24D) and 4-methyl-3-thiosemicarbazide (MT24D) or 4-phenyl-3-thiosemicarbazide (PT24D) |
Ni(II) |
Antibacterial |
[85] |
27. |
N,N’-bis(2-hydroxy-3-R-benzylidene)-1,6-diamino-3-azahexane |
Co(III) |
Antifungal, Antimicrobial Anticancer |
[86] |
28. |
(1,4-phenylenebis(methanylylidene))bis(benzene-1,2-diamine) |
Cu(II), Co(II), Ni(II) |
Antibacterial Antifungal |
[87] |
29. |
5-phenylisoxazole-3-carboxylic acid hydrazide |
V(III) |
Antidiabetic |
[88] |
30. |
5-(4-fluorophenyl)thiophene-2-carboxylic acid hydrazide |
V(III) |
Antidiabetic |
[89] |
Conclusion
Schiff bases are versatile organic ligands and have an array of useful applications such as intermediates, chemosensors, as dyes and pigments and numerous biological properties owing to the presence of azomethine nitrogen. Schiff bases have a potential ability to coordinate with metal atoms belonging to main group, transition metals and rare earths owing to their bi, tri or multidentate nature and structural flexibility. The metal complexes have been studied for their physic-chemical and structural features including catalytic activity. The metal complexes of Schiff bases can move across the membrane through diffusion. Solubility of the Schiff bases along with toxicity are other issues which also need to be addressed.
Acknowledgement
The authors are thankful to Department of Chemistry, Integral University and Department of Chemistry, Isabella Thoburn College for the support.
Conflict of interest
The authors declare they have no competing interest
Funding Sources
None
References
- Song, W.J.; Sontz, P.A.; Ambroggia, X.I.; Tezcan, F.A. Annu. Rev. Biophys. 2014, 43, 409–431
CrossRef - Sigel, H.; Sigel, A.; Z. Für Nat. 2019, 74.
CrossRef - Chellan, P.; Sadler, P.J.; Philos. Trans. A Math. Phys. Eng. Sci. 2015, 373, 56.
CrossRef - Parkin, G.J.; Chem. Educ. 2006, 83, 791–800.
CrossRef - Rubino, J.T.; Franz, K.J. J; Inorg. Biochem. 2012, 107, 129-143
CrossRef - Anis, I.; Aslam, M.; Afza, N.; Iqbal, L.; Noreen, Z.; Hussain, A.; Safder, M.; Int. J. Curr. Pharm. Res. 2013, 5, 48-57
- Rafique, S.; Idrees, M.; Nasim, A.; Akbar, H.; Athar, A.; Biotech. Mol. Bio. Rev. 2010, 5, 38-45
- Lee, K.B.; Wang, D.; Lippard, S.J.; Sharp P.A.; Proceedings of the National Academy of Sci. 2002, 99, 4239-4244
CrossRef - Louie, A.Y.; Meade, T.J.; Chem. Rev. 1999, 99, 2711-2734
CrossRef - Bartkowiak, D.; Stempfhuber, M.; Wiegel, T.; Bottke, D.; Strahlentheapier Onkologie. 2009, 185, 815-820
CrossRef - Marcon, G.; Carotti, S.; Coronnello, M.; Messori, L.; Mini, E.; Orioli, P.; Mazzie, T.; Cinellu, M.A.; Minghetti, G.; J. Med. Chem. 2002, 45, 1672-1677
CrossRef - Orioli, P.; Fontani, M.; Mini. E.; Carroti, S.; J. Med. Chem. 2000, 43, 3541-3548
CrossRef - Zheng, Y.; Sanche, L.; Radiation Res. 2009, 172, 114-119
CrossRef - Lange, T.S.; Kim, K.K.; Singh, R.K.; Strongin, R.M.; McCourt, C.K.; Brard, L.; PLoS One. 2008, 3, e2303
CrossRef - Top, S.; Vessières, A.; Leclercq, G.; Quivy, J.; Tang, J.; Vaissermann, J.; Michel, Huché, M.; Jaouen, G.; Chem.: A European J. 2003, 9, 5223-5236
CrossRef - Baile, M.B.; Kolhe, N.S.; Deotarse, P.P.; Jain, A.S.; Kulkarni, A.A.; Int. J. Pharma. Res. Rev. 2015, 4, 59-66
CrossRef - Shaker, A.M.; Nassr, L.A.E.; Adam, M.S.S.; Mohamed, I.M.A.; J. Korean Chem. Soc. 2013, 5,560
CrossRef - Pascal, P.; Top, S.; Vessieres, A.; Huche, M.; Hillard, E.A.; Salamon, E.; Jaouen, G.; J. Med. Chem. 2005, 48, 2814-2821
CrossRef - Allardyce, C.S.; Dyson, P.J.; Ellis, D.J.; Health, S.L.; Chem. Comm. 2001, 15, 1396-1397
CrossRef - Anne, V.; Micheal, H.; Elizebeth, A.; Hillard, Emmanuel, S.; J. Med. Chem. 2005, 48, 2814-2821
CrossRef - Claire, S.; Allardyce; Paul, J.; Platinum Metal Rev. 2001, 45, 65-69
- Mokhles, M.; Abd Elzaher Ammar, A.; Labib Hanan, A.; Mousa Samia, A.; Moustafa Mamdouh M. Ali.; Ahmed, A.; Rashedy, E. I.; Beni-Suef University J. Basic App. Sci. 2016, 5, 85-96
CrossRef - Bassetti, S.; Hu, J.; Agostino, Jr. R.B.; Sherertz, R.J.; Antimicrob. Agents and Chem. 2001, 45, 1535-1538
CrossRef - Lorisean, P.M.; Carciunescu, D.G.; Doadrio, Villarejo, J.C.; Certad, Fombona, G.; Gayyal, P.; Tropical Med. Parasitol. 1992, 43, 110- 114
- Singh, P.; Singh, V.; Singh, S.; Divya, P.; Tiwari, K.; Mishra, M.; J. Mole. Str. 2014, 1058, 71-78
CrossRef - Kumar, D.; Singh, V. K.; J. Drug Dis.Therapeutic. 2014, 2, 24-32
- Chohan, Z. H.; Met. Based Drugs. 1999, 6, 75-80
CrossRef - Prakash, A.; Adhikari, D.; Int. J. Chem. Tech. Res. 2011, 3, 1891-1896
- Dhumwad, S. D.; Gudasi, K. B.; Gudar, T. R.; Indian J. Chem. 1994, 33A, 320-324
- French, F. A.; Banz, E. J.; J.; Cancer Res. 1966, 26, 1638-1640
- Kumar, S.; Dhar, D. N.; Saxena, P. N.; J. Sci. Ind. Res. 2009, 68, 181-18
- Love, B.E.; Ren, J.; J. Org. Chem. 1993, 58, 5556–5567
CrossRef - da Silva, C.M.; da Silva, D.; da Silva, L.; Modolo, L.V.; Alves, R.B.; Resende, M.A.; Martins, C.; de Fa´ tima, A.; J. Adv. Res. 2011, 2, 1–8
CrossRef - de Souza, A.O.; Galetti, F.C.S.; Silva, C.L.; Bicalho, B.; Parma, M.M.; Fonseca, S.F.; Quim Nova. 2007, 30, 1563–1566.
CrossRef - Shi, L.; Ge, H.M.; Tan, S.H.; Li, H.Q.; Song, Y.C.; Zhu, H.L.; Eur. J. Med. Chem. 2007, 42, 558–564.
CrossRef - Pandeya, S.N.; Sriram, D.; Nath, G.; de Clercq, E.; IL Farmaco. 1999, 54, 624–628
CrossRef - Hearn, M.J.; Cynamon, M.H.; J. Antimicrob. Chemother. 2004, 53, 185–191
CrossRef - Vicini, P.; Zani, F.; Cozzini, P.; Doytchinova, I.; Eur. J. Med. Chem. 2002, 37, 553–564
CrossRef - Rollas, S.; Gulerman, N.; Erdeniz H.; II Farmaco. 2002, 57, 171-174
CrossRef - Sakiyan, L.; Logoglu, E.; Arslan, S.; Sari, N.; Sakiyan, N.; Biometals. 2004, 17, 115-120
CrossRef - Sriram, D.; Yogeeswari, P.; Myneedu, N.S.; Saraswat, V.; Microwaveassisted synthesis and their evaluation of anti-HIV activities. Bioorg. Med. Chem. Lett. 2006, 16, 2127-2129
CrossRef - Kumar, K.S.; Ganguly, S.; Veerasamy, R.; De Clercq, E.; Eur. J. Med. Chem. 2010, 45, 5474-5479
CrossRef - Qin, W.; Long, S.; Panunzio, M.; Biondi, S.; Molecules. 2013, 18, 12264-1228
CrossRef - Rathelot, P.; Vanelle, P.; Gasquet, M.; Delmas, F.; Crozet, M.P.; David, T.; Eur J Med Chem. 1995, 30, 503-508
CrossRef - Bringmann, G.; Dreyer, M.; Faber, J.H.; Dalsgaard, P.W.; Staerk, D.; Jaroszewski, J.W.; J. Nat. Prod. 2004, 67, 743–748
CrossRef - Rehman, W.; Baloch, M.K.; Muhammad, B.; Badshah, A.; Khan, K.M.; Chin. Sci. Bull. 2004; 49, 119–12
- Guo, Z.; Xing, R.; Liu, S.; Zhong, Z.; Ji, X.; Wang, L.; Carbohydr. Res. 2007, 342, 1329–1332
CrossRef - Karthikeyan, M.S.; Prasad, D.J.; Poojary, B.; Bhat, K.S.; Holla, B.S.; Kumari, N.S.; Bioorg. Med. Chem. 2006, 14, 7482–7489
CrossRef - Echevarria, A.; Nascimento, M.G.; Geroˆ nimo V.; Miller, J.; Giesbrecht, A.; J Braz Chem. Soc. 1999, 10, 60–64
CrossRef - Pandeya, S.N.; Sriram, D.; Nath, G.; de Clercq, E.; IL Farmaco. 1999, 54, 624–628
CrossRef - Arunadevi, A.; Raman N.; J. Coord. Chem. 2020, 73, 2095-2116
CrossRef - Zayed, E.M.; Zayed, M.A.; Spectrochim. Acta Part A: Mol. Biol. Spect. 2015, 143, 81-90
CrossRef - Alam, M.S.; Choi, J.H.; Lee, D.U.; Bioorg. Med. Chem. 2012, 20, 4103-4108
CrossRef - Munjal, M.; J. Pharmacog. Phytochem. 2017, 6, 354-360
- Maity, D.; Int. J. Res. Anal. Rev. 2019, 6, 471-478
- Chang, X.; Wang, J.; Jiao, C.; Li, R.; Yang, P.; Liu, Q.; Chem. Eng. J. 2012, 198, 412-419
CrossRef - Li, L.J.; Wang, C.; Tian, C.; Yang, X.Y.; Hua, X.X.; Du, J.L.; Res. Chem. Intermed. 2013, 39, 733-746
CrossRef - Tofazzal M.; Tarafder H.; Ali, M.A.; Saravanam, N.; Weng, W.Y.; Kumar S.; Umar Tsafe N.; Crouse, K. A.; Trans. Met. Chem. 2000, 25, 295-298
CrossRef - Thomas, A. B.; Nanda, R. K.; Kothapalli, L. P.; Hamane, S. C.; Arabian J. Chem. 2016, 9, S79-S90
CrossRef - Bhat, M. A.; Al-Omar, M.A.; Acta Poloniae Pharmaceutica. 2011, 68, 375–380
- Kulkarni, A. A.; Wankhede, S. B.; Dhawale, N. D.; Yadav, P. B.; Deore, V. V.; Gonjari, I. D.; Arabian J. Chem. 2012, S1878535212001797
- Aly, M. M.; Mohamed, Y. A.; El-Bayouki, K. A. M.; Basyouni, W. M.; Abbas, S. Y.; Euro. J. Med. Chem. 2010, 45, 3365–3373
CrossRef - Zahan, R.; Ahmed, S.; Sharmin, T.; Halim, M.A.; Rahi, M.S.; Md. Chanmiya Sheikh, C.; et al. App. Organomet. Chem. 2020, 35, 1-7
CrossRef - Parekh, H. M.; Mehta, S. R.; Patel, M. N.; Russ. J. Inorg. Chem. 2006, 51, 67-72
CrossRef - Munteanu, C.R.; Suntharalingam, K.; Dalton Trans. 2015, 44, 13796–13808.
CrossRef - Mjos, K.D.; Orvig, C.; Chem. Rev. 2014, 114, 4540–4563
CrossRef - Shanker, K.; Rohini, R.; Ravinder, V.; Reddy, P. M.; Ho, Y. P.; Spect. Acta Part A: Mol. Biomol. Spect. 2009, 73, 205-211
CrossRef - Yuan, C.; Gao, L. X.; Guo, W. M.; Fu, L. X.; Zhu, M.; J. Bio. Inorg. Chem. 2009, 14, 841-851
CrossRef - Zhang, X.; Wang, J.; Jiao, C.; Li, R.; Yang, P.; Liu, Q.; et al. Chem. Eng. J. 2012, 198, 412-419
CrossRef - Zhou, G.P.; Hui, Y.H.; Wan. N.N.; Liu, Q.J.; Xie, Z.F.; Wang, J.D.; Chin. Chem. Lett. 2012, 23, 690.
CrossRef - Kumar, S.; Dhar, D. N.; Saxena, P. N.; J. Sci. Ind. Res. 2009, 68,181-187
- Singh, D.; Singh, R. V.; Goyal, R. B.; App. Organomet. Chem. 2004, 18, 73-82
CrossRef - Li, L. J.; Wang, C.; Tian, C.; Yang, X. Y.; Hua, X. X.; Du, J. L.; Res. Chem. Intermed. 2013, 39, 733-746.
CrossRef - Nair, M. S.; Arish, D.; Joseyphus, R. S.; J. Saudi Chem. Soc. 2012, 16, 83-88
CrossRef - Rayati, S.; Rafiee, N.; Wojtczak, A.; Inorg. Chim. Acta. 2012, 386, 27-35
CrossRef - Singh, B. K.; Mishra, P.; Prakash, A.; Bhojak, N.; Arabian J. Chem. 2017, 10, 472-483
CrossRef - Yousif, E.; Majeed, A.; Al-Sammarrae, K.; Salih, N.; Salimon J.; Abdullah, B.; Arabian J. Chem. 2017,10, 1639-1644
CrossRef - Zafar, H.; Ahmad, A.; Khan, A. U.; Khan, T. A.; J. Mol. Str. 2015, 1097, 129-135
CrossRef - Selvaganapathy, M.; Raman, N.; J. Chem. Bio. Therapeut. 2016, 01,
CrossRef - Wani, M. Y.; Ahmad, A.; Malik, M.A.; Med. Chem. Res. 2016, 25,2557-2566
CrossRef - Tyagi, P.; Tyagi, M.; Agrawal, S.; Chandra, S.; Ojha, H.; Pathak, M.; Spectrochimica Acta. 2017, 171,246-257
CrossRef - Vinusha, H.M.; Shiva Prasad Kollur, H.D.; Revanasiddappa.; et al. Results in Chemistry, 2019, 1, 100012.
CrossRef - Klamm, B. E.; Windorff, C. J.; Celis-Barros, C.; Marsh, M.L.; Meeker, D.S.; Albrecht-Schmitt, T.E.; Inorg. Chem. 2018, 57, 15389-15398
CrossRef - Jai Devi J.; Batra, N.; Spectrochim. Acta Part A: Mol. Biomol. Spect, 2015, 135, 710-719.
CrossRef - Nurul, N.M.; Ishak, Jamsari J.; et al. J. Mol. Str., 2019, 1198, 126888.
CrossRef - Diab, M. A.; Mohamed, G. G.; Mahmoud, W.H.; El-Sonbati, A.Z.; Morgan S.M.; Abbas, S.Y.; App. Organomet. Chem. 2019, e4945
- Xiang Liu; Jean-René Hamon; Coord. Chem. Rev. 2019, 389, 94-118
CrossRef - Saranya, J.; Kirubavathy S. J.; Chitra, S.; Arabian J. Sci. Eng., 2020, 45, 4683-4695
CrossRef - Szklarzewicz, J.; Jurowska, A.; Matoga, D.; Kruczała, K.; Kazek, G.; Mordyl B.; Papież, M.; Polyhedron. 2020, 185, 114589
CrossRef
This work is licensed under a Creative Commons Attribution 4.0 International License.