Analysis of Solute-solvent Interactions of l-isoleucine in Aqueous KCl and NaCl Solutions Across Varying Temperatures
N. S. Pathan*, U. P Manik
and P. L. Mishra
Department of Physics, S.P. College, Maharashtra, India.
Corresponding Author E-mail:nehapatan0204@gmail.com
DOI : http://dx.doi.org/10.13005/ojc/400604
Article Received on : 16 Aug 2024
Article Accepted on :
Article Published : 28 Nov 2024
Reviewed by: Dr. Paritosh Mishra
Second Review by: Dr. Ajit Kumar Maddheshiya
Final Approval by: Dr. Ioana Stanciu
Physicochemical and volumetric properties of of L-isoleucine (ISL) have been studied in the presence of two electrolytes, potassium chloride (KCl) and sodium chloride (NaCl), at temperatures of 283.15K and 293.15K. The empirically measured physical properties such as density and speed of sound have been utilized for the computation of several parameters such as isothermal compressibility, relative association, non-linier parameter, surface tension, adiabatic compressibility etc., These parameters are interpreted in the context of molecular interactions. Because of the structure-making ability of salt ions and the electrostriction of the solvation shells, it is discovered that the compressibility of the solutions and the solvent decrease with an increase in electrolyte concentration and l-isoleucine amount. The obtained parameters indicate that there is a substantial ion-solvent interaction present in the solution. The results underscored the enhanced ion-solvent interactions, ion-pair formation, and structure-stabilizing effects of L-isoleucine in the presence of KCl/NaCl.
KEYWORDS:ions.; L-Isoleucine; Potassium Chloride; Sodium Chloride
Download this article as:
Copy the following to cite this article: Pathan N. S, Manik U. P, Mishra P. L. Analysis of Solute-solvent Interactions of l-isoleucine in Aqueous KCl and NaCl Solutions Across Varying Temperatures. Orient J Chem 2024;40(6). |
Copy the following to cite this URL: Pathan N. S, Manik U. P, Mishra P. L. Analysis of Solute-solvent Interactions of l-isoleucine in Aqueous KCl and NaCl Solutions Across Varying Temperatures. Orient J Chem 2024;40(6).Available from: https://bit.ly/3Vu7YUS |
Introduction
Taking place in the living world: “protein”. When it comes to supporting diverse reactions in biological systems, proteins are nonpareil among all types of biomolecules. All living things use proteins for a variety of biological processes, including catalysis, transcription, DNA replication, storage function, etc.,1,2. Proteins, essential involvement in this process can be comprehended only via an awareness of their surroundings. Most proteins are found in water and are compound-rich which affects their structure and characteristics including stability, solubility, enzymatic activity, and dissociation3,4. One element unites numerous biological processes Because of their complex molecular structures, proteins are difficult to directly investigate and take a lot of time. One approach that reduces complexity and calls for simpler testing methods is to examine the interaction in systems that include model molecules like amino acids and peptides. Of all the chemical compounds proteins are the biomolecule found in the highest amount of all living things. The most critical biological functions such as cell development and maintenance, movement, and resistance depend on them5-7.
Proteins are intricate structures, and a variety of distinct interactions control how they behave in solutions8. Amino acids can be employed for investigations that are anticipated to have an impact on the structure and conformation of proteins because they are the low-molecular-mass model compound that forms the building blocks of protein9. Low molecular weight compounds are crucial to study because they can be used to systematically change the structure, allowing for simple identification of the side chain group of peptides and amino acids10,11. Understanding ionic solvation or ion association can help you better understand how ionic liquids are used in current technology. Proteins, Characteristics, and structure are greatly impacted by electrolytes12,13. The ionic association of the electrolytes in the solution is determined by the mechanism of the ion’s salvation, which in turn depends on the make-up of the solute-solvent combinations14. Because of their numerous applications in the biosciences, food, cosmetics, medication administration, detergency, and biotechnology, electrolytes play a crucial role in the solubility of amino acids. The need to understand more about how proteins, amino acids, and electrolytes interact has grown in recent years 15-17. The acoustic and volumetric the solute’s noteworthy hydration capabilities were uncovered through study of amino acids in water-based solutions. The majority of these interactions, including electrostatic, hydrophobic, and hydrogen-bonding interactions, are non-covalent18,19. The investigation of these interactions can provide a crucial understanding of the stability of the structure and unfolding behavior of proteins with globular structures20.
Investigation of the acoustical and volumetric studies on the characteristics of ionic liquids and amino acids. However, there is a noticeable lack of comprehensive investigation of the volumetric and transport properties of L-isoleucine in electrolyte solutions. Now, an effort is being made to comprehend the interactions between salt and L-isoleucine within molecules. Here the density as well as ultrasonic speed data for the L-isoleucine in an aqueous solution was tested at temperatures T (283.15 and 293.15) K, containing 0.1 mol kg-1 of NaCl and KCl. To understand the characteristics and relative potency of several types of interaction (Viz ion solvent, solute-solvent) interaction various volumetric and thermos-acoustical parameters are calculated, such as Acoustic impedance, surface tension, nonlinear parameters, relaxation strength, isothermal compressibility, adiabatic compressibility, and relative association have also been examined.
Materials and Methods
The AR-grade chemicals employed in this study consist of L-isoleucine, sodium chloride (NaCl), and potassium chloride (KCl), all sourced from Hi-Media Private Limited with a minimum purity of 99.9%. Detailed information regarding these chemicals is provided in Table 1. The binary solutions of L-isoleucine were dissolved in a solution of potassium chloride and sodium chloride, with a concentration of 0.01 mol/Kg of water. To eliminate unnecessary errors, all glassware was thoroughly cleaned with freshly double distilled water and acetone and then dried thoroughly before use. A digital ultrasonic velocity interferometer with an overall accuracy of ±0.001m/s was utilized to measure the ultrasonic velocity operating a frequency of 2 MHz provided by (VI Micro System Pvt. Ltd., Chennai). A quartz crystal that had been stimulated by a radio frequency oscillator was a source of the ultrasonic wave. The desired solution was added to the cell at a steady temperature and circulated inside the outer jacket. The densities of the solutions were determined and a 10 ml specific gravity density bottle with an accuracy of ±2×10-2 kg/m3 and electronic weighing balance (CONTECH CA-34) with an accuracy of ±0.0001 g. The experiment’s temperature was kept constant by using an automatic thermostatic water bath, which has a temperature accuracy of ±1K. Using measured values of density and velocity, the following volumetric and acoustical parameters have also been determined.
Table 1: Details of the materials utilized
Compound |
Source |
CAS No. |
Molecular weight (g/mol) |
Mass Fraction Purity |
L- isoleucine |
Hi. Media. Pvt. Ltd. |
73-32-57 |
131.17 |
99.9% |
Potassium Chloride |
7447-40-7 |
74.55 |
||
Sodium Chloride |
7647-15-5 |
58.44 |
Results and Discussion
Measurements were conducted to determine the densities and ultrasonic velocities of L-isoleucine under two different different temperatures (283.15K and 293.15K) in aqueous solutions of KCl and NaCl. Molality measurements were made to ascertain the content of the potassium chloride and sodium chloride solutions used as solvents. The values in Figures 1 and 2 shows the concentration of L-isoleucine rises with temperature in both KCl and NaCl solutions, it becomes clear that the measured density values of the aqueous binary system also increase. The travel of ultrasonic waves in solution can be influenced by factors such as temperature and concentration21. Sound speed increases as a result of the cohesiveness that comes from ionic hydration and the formation of hydrogen bonds between L-isoleucine, water, and salts. Hydrogen atoms are compelled to come together during the ultrasonic wave’s compression cycle, which causes the energy to be absorbed and partially irreversibly break hydrogen bonds.22. A more compact structure is created when the amino acid molecule and water molecules interact. This serves as a material conduit via which sound waves can travel.
![]() |
Figure 1: Velocity with various concentrations and temperatures. |
![]() |
Figure 2: Density with various concentrations and temperatures |
The speed of the sound wave and the density of the medium define acoustic impedance. It is the ratio of the instantaneous pressure at any particle of the medium to the instantaneous velocity of that particle. When the temperature and concentrations of solutes change, the behavior of Z tends to follow the same pattern as the behavior of the ultrasonic velocity23.Using density and ultrasonic velocity acoustic impedance is calculated using equation 1. As seen in equation 1 where velocity is U and density is ρ.
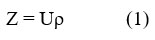
The relationship between acoustic impedance (z) and temperature/concentration is illustrated in Figure 3, the observed rise in acoustic impedance appears to correlate with an apparent dissociation of repulsive forces as temperatures increase24. The tightening of molecules at higher concentrations is indicated by the rise in acoustic impedance with molarity. The effective solute-solvent interaction is responsible for the increase in acoustic impedance25. The surface tension variations of L-isoleucine in water and aqueous solutions of KCl and NaCl are displayed in Figure 4, along with the surface tension values determined by Equation 2.

![]() |
Figure 3: Surface Tension with various concentrations and temperatures |
![]() |
Figure 4: Non-linear parameters with various concentrations and temperatures |
Surface tension is a phenomenon that refers to the cohesive forces experienced by molecules in a liquid’s thin surface layer. As a starting point, surface tension can be used to determine whether intermolecular forces are present21-23. As the temperature increases and the intermolecular interaction between the liquids intensifies, Additionally, at different temperatures, the surface tension values increases with the concentration of L-isoleucine and decrease via lower temperatures.The findings presented in this study offer valuable insights into the complex dynamics of solute-solvent interactions and the behavior of salt and amino acids in micellar systems. 23,24 The Hartmann-Balizar formulation, as stated in equation 3, provides a suitable representation for non-linear parameters (B/A) and has been utilized to determine B/A values using sound velocity.

According to Hartmann-Balizar Theoretically, intermolecular potential energy controls the sound speed and how it varies in liquids. Several theoretical methods have been established to determine nonlinearity parameters (B/A) for liquids that are pure and liquid mixtures The B/A reviews for the liquids have been interpreted as a measurement of their hardness. The interaction between compounds in binary systems is the focus of these values 15,19. Figure 5 makes it clear that at lower concentrations, there is a greater interaction between L-isoleucine and water in a binary combination19. Equation 4 is employed to calculate the (KT) of L-isoleucine in aqueous solutions of KCl and NaCl across various temperatures.
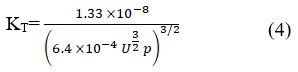
![]() |
Figure 5: Relaxation Strength with various concentrations and temperatures |
The main trends in isothermal compressibility (KT) between 283.15K and 293.15K are shown in Figure 6. It has been noted that, irrespective of the relation applied, the expected (KT) values of fall as concentration increases21. The observed “KT” values appear to be caused by a decrease in the average kinetic energy and free volume of each individual solute-solvent molecule in the amino acid solution. This is because the concentration of L-isoleucine in each of the three solvents increases with an increase in KT values. B/A variation with concentration has a nearly linear relationship. It shows that there was no complicated development between the mixture’s components. The formation of interaction in the case of L-isoleucine in the presence of sodium chloride and potassium chloride has been deduced from the B/A values. Temperature-dependent value of relaxation strength can be defined by equation 5.

Where U is the ultrasound velocity of the mixture and U∞ is continual and has a volume of 1600m/sec The relaxation strength is a complete measure of interaction strength and indicates that there is more interaction between the solutions of (L-isoleucine + water) and (L-isoleucine + water + NaCl/KCl). As temperature and concentration rise, the relaxation strength falls (as shown in Figure 5), indicating that there is solute-solvent interaction present in the system. The comparatively lower relaxation strength values that are shown as concentration increases serve as evidence for this.
![]() |
Figure 6: Isothermal Compressibility with various concentrations and temperatures |
![]() |
Figure 7: Adiabatic compressibility with various concentrations and temperatures |
The results of adiabatic compressibility (β) illustrated in Figure 7 demonstrate that, in all aqueous solvent solutions, adiabatic compressibility decreased with increasing solute content. 23The values of adiabatic compressibility of L-isoleucine in aqueous electrolyte solutions have been calculated using equation 6.
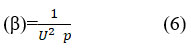
The computation made using the velocity data is supported by the decreasing adiabatic compressibility seen for L-isoleucine in water, isoleucine + NaCl, and isoleucine + KCl. Since water experiences increased electrostrictive compression of the solute molecules, solutions are substantially less compressible. As the new entities created as a result of molecular associations grow more compact and less compressible, the molecular interactions in these systems may be improved as the solute content rises when additional hydrogen bonds are established between the molecules of the solute and the solvent, the compressibility experienced a noticeable increase. To decrease. The compressibility of L-isoleucine in KCl decreases more significantly compared to other solvents, indicating that KCl exhibits a higher potential for molecular interaction. With an increase in solution concentration, there is a rise in adiabatic compressibility14,19. This may be explained by the fact that solvent molecules are more concentrated close to ions, which leads to weak ion-solvent contact. This proposes the interaction between the solute and solvent.
The relative association parameters evaluate the non-ideality of the solution and provide information about the numerous molecular interactions occurring within the solution. Two opposing elements mostly influence the relative association. The relative association of the examined solutions (L-isoleucine + water), (L-isoleucine + water +KCl), and (L-isoleucine + water + NaCl) increases with temperature and increases as L-isoleucine content increases17,18. Increasing temperature led to a decreased density of the solutions generally. RA of L-isoleucine in the examined solutions was calculated using equation 7.
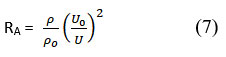
Structure collapses and related solvent molecules lose relative connection when a solute is given to them relative association (RA). Using free solvent molecules to dissolve the solute promotes relative association. At all studied temperatures, relative association (RA) rises linearly as solution concentration rises. The graph shown in Figure 8 illustrates the relationship between relative association (RA) and molar concentration in the mixture of L-isoleucine and electrolytes. The increase in relative association values that occurs with an increase in salt concentration shows that the solute’s solvation takes precedence over the solvent structure collapsing, which would otherwise increase the solute’s solvation by free solvent molecules15,19,20,25. Additionally, it has been found that as temperature increases, relative association (RA) values for L-isoleucine solutions fall. The molecule’s increased thermal mobility may be to blame for the drop in relative association with a temperature rise, which causes the associated solvent and solute molecules to separate, impending the relative association.
![]() |
Figure 8: Relative Association with various concentrations and temperatures |
Conclusion
Volumetric, acoustic, and thermodynamic measurements to explore the dynamics between L-isoleucine and two electrolytes, NaCl and KCl, within aqueous solutions. The positive value of relative association (RA) and acoustic impedance (Z) alongside L-isoleucine concentration in each solvent, coupled with the impact of temperature on solvation, underscores a reduction in solvation due to thermal agitation. This phenomenon leads to the liberation of solvent molecules from the solute, diminishing solvation. Strong interactions between solute-solvent molecules were noted, suggesting fewer free molecules within the solvent. The data interpretation suggests significant molecular association in liquid mixtures of L-isoleucine, particularly at temperatures of 283.15K and 293.15K. Notably, the presence of an additional hydroxyl group in L-isoleucine + KCl resulted in more pronounced solute-solvent interactions compared to L-isoleucine + NaCl.
Acknowledgment
I am expressing our deepest gratitude and appreciation for the assistance and support received throughout the completion of this research paper. I would like to thank Neha S. Pathan, Dr. U.P. Manik and Paritosh L. Mishra for her invaluable guidance, encouragement, and support throughout this research endeavour.
Conflict of Interest
The authors declare that there is no conflict of interest regarding the publication of this article.
Funding Sources
The author(s) received no financial support for the research, authorship, and/or publication of this article.
Data Availability Statement
Information can be accessed upon request.
Author Contributions
Neha S. Pathan: Research, formal analysis, data curation, and drafting of early drafts.
Urvashi P. Manik: creativity, methodology, draft creation, editing, and oversight.
Paritosh L. Mishra:Ideas, guidance, techniques, proofreading, and composition assessment.
References
- Z. Yan, X. Chen, L. Liu, and X. Cao, J. Chem. Thermodyn., 2019,138, 1–13.
CrossRef - F. Kooshkebaghi, M. Jabbari, and A. Farajtabar, J. Mol. Liq., 2024,124229.
CrossRef - J. Gupta, A.K. Nain and D. Chand, Org. Med. Chem. Int. J., 2020,9, 66-85.
- A. Hirano, M. Wada, T. K. Sato, and T. Kameda, Int. J. Biol. Macromol., 2024, 261, 129724.
CrossRef - M. Asghar, A. Sohail, M. Muneer, G. Abbas, S. Shah, and T. Ahmad, J. Mol. Liq., 2024, 398, 124331.
CrossRef - S. Baluja and R. M. Talaviya, J. Chem. Eng. Data, 2016, 61, 4, 1431–1440.
CrossRef - H. Kumar, G. Singh, R. Kataria and S.K. Sharma, J. Mol. Liq., 2020, 303, 112592.
CrossRef - T. Brindha, R. Rathinam, S. Dheenadhayalan, M. Sunitha and V. Gokila, Rasayan J. 2023, Chem., 16.
- M. Kaur, Orient. J. Chem., 2021 37, 3, 722–734.
CrossRef - S. Sekhar, B. Nanda, S. Ranjan, B. Dalai, and B. B. Nanda, J. Mol. Liq., 2022, 351, 118644.
CrossRef - B. Naseem, I. Arif, and M.A. Jamal, Arab. J. Chem., 2020, 13, 7759.
CrossRef - P. Tekade, B. Tale and S. Bajaj, Russ. J. Phys. Chem. A, 2018, 92, 2679.
CrossRef - P.L. Mishra, A.B. Lad and U.P. Manik, J. Sci. Res., 2021, 65, 72.
CrossRef - S. Noshadi and R. Sadeghi, Phys. Chem. Res., 2018, 6, 3, 531–546.
- R. Rathinam, T. Brindha, S. Dheenadhayalan and M. Sunitha, Asian J. Chem., 2023, 35, 1291.
CrossRef - R. Ravichandran and C. Senthamil Selvi, Rasayan J. Chem., 2014, 7, 246.
- S. Singh, M. Talukdar and U.N. Dash, J. Mol. Liq., 2018, 249, 815.
CrossRef - P. Zheng, D. Cai, J. Zhao, and W. Shen, J. Chem. Thermodyn., 2017, 113, 394–398.
CrossRef - D.H. Dagade, S.P. Shinde, K.R. Madkar and S.S. Barge, J. Chem. Thermodyn., 2014,79, 192.
CrossRef - S.P. Neha, P.M. Urvashi and L.M. Paritosh, Mater. Today Proc. 2023.
CrossRef - Zhang, Y. Zhao, A. Zang and A. Long, Rock Mech. Rock Eng., 2024,57, 1287.
CrossRef - T. Brindha, R. Rathinam, S. Dheenadhayalan, S. Supriya, Asian J. Chem., 2022, 34, 3320.
CrossRef - R. Thakur, R. Sharma, A. Kumar, Orient. J. Chem., 2015, 31 363-369.
CrossRef - B. Kanagarajan, S. Parveen, R. Ramasamy and U. Ramasamy, Bull. Chem. Soc. Ethiopia, 2023, 37, 623.
CrossRef - N. S. Pathan, U. P. Manik, and P. L. Mishra, Mater. Today, Proc., 2024.
This work is licensed under a Creative Commons Attribution 4.0 International License.