Ultrasonic Characterization on Glycine Dissolve in Saline Salt (MgSO4 andNa2SO4) Solution in View to Sustainable Farming
Sonali V. Hepat*and Urvashi P. Manik
Department of Physics, S. P. College, Chandrapur, Maharashtra, India.
Corresponding Author E-mail:sonalihepat1992@gmail.com
Article Received on : 09 Oct 2024
Article Accepted on :
Article Published : 28 Nov 2024
Reviewed by: Dr. Jyothi Nimmagadda
Second Review by: Dr. Asif Ali
Final Approval by: Dr. Bal krishan Sharma
Soil salinity is caused by the increased concentration of calcium, magnesium, sodium, and potassium ions in the soil. Salinity alters the physiological characteristics of soil and inhibits plant’s ability to absorb water and other nutrients. Numerous studies have shown that amino acids have a bio stimulatory effect on plant growth and improved nutrient uptake. In this work a strategy has been developed to cut the salinity problem by using ultrasonic technique. For this, the impact of Glycine on soil salinity has been studied. The ultrasonic velocity and density of Glycine in water as well as 0.2NMagnesium sulphate and Sodium sulphate solution as function of concentration at different temperatures (313.15 and 318.15k) have been determined. By using this basic data different thermo-physical properties of mentioned systems have been calculated. Thus, present scenario confirms the formation of stronger interaction between molecules of saline salt and Glycine. Furthermore, stronger interaction forms strong hydrogen bond hence, Glycine is effective in view to reduce the salinity.
KEYWORDS:Glycine; Hydrogen Bonding; Salinity; solute-solvent interaction; Ultrasonic velocity
Download this article as:
Copy the following to cite this article: Hepat S. V, Manik U. P. Ultrasonic Characterization on Glycine Dissolve in Saline Salt (MgSO4 andNa2SO4) Solution in View to Sustainable Farming. Orient J Chem 2024;40(6). |
Copy the following to cite this URL: Hepat S. V, Manik U. P. Ultrasonic Characterization on Glycine Dissolve in Saline Salt (MgSO4 andNa2SO4) Solution in View to Sustainable Farming. Orient J Chem 2024;40(6). Available from: https://bit.ly/419FByG |
Introduction
The thermoacoustic and volumetric properties of amino acids gives important information regarding type interaction between constituent molecules and stability of proteins.1 ultrasonic velocity, density and other thermodynamic parameters helps to understand physiochemical properties of liquid mixture like molecular association, dissociation, structural changes associated with liquid mixture.2,3 the physiological properties can be derived from obtained experimental data and allow to modify desired properties of solvent for special application.4-6
Plants requires soil medium for their development. Sustainable agriculture is farming in which amending something to improve the soil fertility with less harmful environmental effects with rise in crop yield. it reduces environmental pollution.7 The higher concentration of salts like sodium, calcium, potassium leads to the salty soil. salinity of soil affects the physiological properties of plants. Salinity of soil affects the plants growth and biochemical properties of soil. The use of fertilizers containing K, NH4, P are effective and covers the adverse effect of salinity.8 The world population is still growing and to feed such large population, we must concentrate on land under production and bringing non-arable and less productive land under production. The soil salinity affects formerly arable land and resulting in to removal from land under production. Many researchers have studied the impact of soil salinity on nutrient composition of plant. The negative effect of soil salinity on plant growth and development arises due to ionic imbalance.9-11 A significant amount of costly and harmful fertilizers is typically used in agricultural production to provide plants with necessary nutrients for a limited duration. The majority of applied fertilizers responsible for environmental pollution and degradation of the ecosystem. Efficient utilization of nutrients from fertilizers is crucial for meeting environmental standards and addressing economic concerns. By using sustainable methods, it is feasible to increase the biological uptake of nutrients by plants by applying amino acids or incorporating microorganisms.12,13 Application of amino acids can improve the vegetative, reproductive, and quantitative characteristics of plants, among other aspects of their growth. Their beneficial impacts on plant growth were enhanced under salt stress conditions. amino acids are great alternatives for biofortifying agricultural crops along with replacing chemical fertilizers.14,15
Understanding proteins’ structural and functional features requires an understanding of their aqueous solution stability. In this work, amino acids in aqueous salt solutions are studied thermodynamically. As a function of temperature (313.15, 318.15 K) and solute concentration, the ultrasonic speeds (u) and density (ρ) of glycine in 0.2M aqueous Na2SO4 and MgSO4 solutions are reported. from the obtained data of ultrasonic speed and density various thermodynamic parameters has been calculated in view to understand the type of interaction.
Materials and Methods
All chemicals used in experiment such as Glycine and sodium sulphate and magnesium sulphate are SR grade and AR grade with purity ≥99% were purchased from Himedia.
Name of compound |
Molecular weigth |
Volume |
Molality (Mole) |
Glycine |
119.14g.mol-1 |
30ml |
0-0.2 |
Sodium sulphate |
142.04g.mol-1 |
350ml |
0.2 |
Magnesium sulphate |
246.47g.mol-1 |
350ml |
0.2 |
The Weight of chemical was determined by following formula.

Preparation of Systems
Glycine + H2O
Glycine + H2O +Sodium Sulphate
Glycine + H2O +Magnesium Sulphate
Apparatus
Ultrasonic interferometer operating on 2MHz along with water bath maintained at desired temprature.
10ml Density bottle and weighing balance was used for determination of density.
The 0.2 M aqueous Na2SO4 and 0.2 M aqueous MgSO4 stock solution was prepared in double distilled water. It is used as solvent for preparation of desired experimental solution. The concentration of Glycine is varying over the range 0.02-0.2M. The specific conductivity of doubly distilled water used was less than 18· 10−6 Ω−1 cm−1. all the solutions were stored in airtight bottle to keep away from dissipation and explosion to air. An ultrasonic interferometer (Mittal-M-77, India) is measured at 2MHz with varying temperature. It is based on variable path principle. The average of 20values is taken as final measured value of ultrasonic velocity. The thermostat is used to maintained the temperature of water circulating through insulated surrounding of brass around quartz crystal and cell. A thermostated water bath and ultrasonic interferometer was used to measure ultrasonic velocity. The instruments were keeping at experimental temperature for 30 minutes before recording. The densities of water and experimental solution were carried with 10ml density bottle and electronic mass balance. Electronic balance having the accuracy of ±0.01gm. The average of Three readings of density was taken as calculated density of solution. All the glassware and cell were cleaned with acetone before experiment and instrument is calibrated with double distilled water. The variation in thermodynamic parameter provides crucial information regarding intermolecular forces existing in solution. The ultrasonic velocity measurement of solution serves as the powerful probe to understand physio-chemical properties of solution.
The ultrasonic velocity and density values are utilized in the following parameter computations
Defining Relations
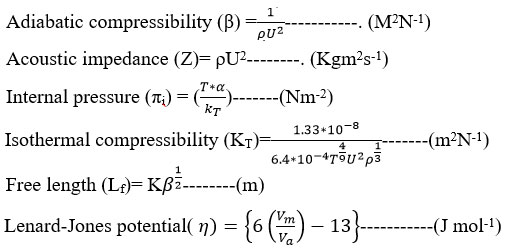
Results and Discussion
The ultrasonic velocity and density of 0.2M MgSO4 and Na2SO4 solution has been measured as function solute concentration of Glycine at a temperature T= 313.15, 318.15K. the measurement of ultrasonic velocity and density useful to calculate various parameters.
Ultrasonic Velocity(U)
From Fig:-01, The ultrasonic velocity increases with increase in concentration of Glycine. The increase in velocity with concentration of solution changes the structure of solution resulting in hydrogen bond formation. The ultrasonic velocity of solution attributed to increase of cohesion brought by dipole-induced dipole interaction or may be due to hydrogen bonding. The value of U is increases with temperature suggests cohesion in molecules due to thermal rupture of structure of water. 15-20
![]() |
Figure 1: Ultrasonic velocity of Glycine with H2O, Na2SO4+ H2O and MgSO4+ H2O at 313.15K and 318.15k. |
Density (ρ)
From Fig-02, the density of solution is goes on increasing with increase in concentration of solute. The increase in density is appears due to hydrophobic properties of solute leads to the hydrogen bond formation. The strong intermolecular forces in solution attributed to cluster formation by solute. The formation of hydrogen due to change in structure of solution leads to decrease in intermolecular free length. 15-21
![]() |
Figure 2: Density of Glycine with H2O, Na2SO4+ H2O and MgSO4+ H2O at 313.15K and 318.15k. |
Adiabatic Compressibility (β)
From Fig-03, The values of adiabatic compressibility decrease with increase in concentration of glycine due to electrostriction compression of solute around the molecule. When an ion enters the solvent environment, due to electrostrictive forces bulk solvent attracted towards ion. Hence a smaller number of ions are available for next incoming ion called compression. The electrostrictive forces cause breaking of water molecule and reduces the compressibility and compact packing of water molecule surrounds the solute. Therefore, decrease in adiabatic compressibility indicates strong solute – solvent interaction. 18-22
![]() |
Figure 3: Adiabatic compressibility of Glycine with H2O, Na2SO4+ H2O and MgSO4+ H2O at 313.15K and 318.15k |
Acoustic Impedance(Z)
The ration of instantaneous pressure excess on any particle of medium is called acoustic impedance. Acoustic impedance depends upon temperature and concentration of solution. As the acoustic wave passes through solution, pressure varies from one particle to another particle. The increase in acoustic impedance with solute concentration suggests increase in instantaneous pressure at molecule. From Fig-04, it is observed that acoustic impedance increases with increase in concentration and temperature suggests strong solute- solvent interaction and internal pressure among the constituent particles is increasing. 20-22
![]() |
Figure 4: Acoustic impedance of Glycine with H2O, Na2SO4+ H2O and MgSO4+ H2O at 313.15K and 318.15k. |
Internal Pressure(πi)
The measurement of cohesive or adhesive forces in solution is called internal pressure. The increase in internal pressure suggests increase in cohesive foresees. The increase in cohesive forces along with greater association in solute-solvent molecules is indicative of decrease in free volume. decrease in free volume suggests closed packing and lesser void space. From Fig-05, increase in internal pressure with increase in temperature and solute concentration suggests strong association in solute-solvent molecules hence molecules are closely packed.21,22
![]() |
Figure 5: Internal Pressure of Glycine with H2O, Na2SO4+ H2O and MgSO4+ H2O at 313.15K and 318.15k. |
Isothermal Compressibility (KT)
isothermal compressibility is relative change in volume with change in pressure at constant temperature. The decrease in isothermal compressibility results in corresponding decrease in free volume and clustering of molecules. High compressibility tends to have low packing density. From Fig-06, it is clear that, isothermal compressibility is decreasing with rising molar concentration of glycine and temperature. It suggests decrease in free volume and molecules are closely packed. It tends to have high packing density.19-21
![]() |
Figure 6: Isothermal compressibility of Glycine with H2O, Na2SO4+ H2O and MgSO4+ H2O at 313.15K and 318.15k. |
Free Length (Lf)
The study of free length is useful to understand intermolecular interactions. Free length is depending upon adiabatic compressibility. When the value of free length is long then free volume is large and decreasing the free available space. The reduction in free length with increase in Glycine concentration and temperature prevails those strong intermolecular interactions among constituent molecules.it supports structure promoting behavior, close packing and stronger association in distinct Molecules by hydrogen bonding. From Fig-07, we conclude that, free length is decreases with increase in temperature and concentration of glycine indicative of close packing of molecules.17-21
![]() |
Figure 7: Free length of Glycine with H2O, Na2SO4+ H2O and MgSO4+ H2O at 313.15K and 318.15k. |
Lenard -jones Potential(η)
Lenard jones potential define the potential energy of interaction among to non-bonding molecules. It is helpful in determination of type of force acting in solute-solvent molecules. It is the larger value of Lenard jones potential suggests presence strong attractive forces between constituent molecules and lower value of η suggest weak attractive forces. From Fig-08, we conclude that, the value of n is boosting withtemperature and molar concentration of Glycine. The increment in value of η indicates increase in force of attraction between molecules. The force of attraction is dominant than repulsive forces.20-23
![]() |
Figure 8: Lenard – Jones potential of Glycine with H2O, Na2SO4+ H2O and MgSO4+ H2O at 313.15K and 318.15k. |
Conclusion
Increase in internal pressure supports increase in cohesive forces and strong association in solute-solvent molecules leads to closed packing of molecules.
The decrease in adiabatic compressibility decreases in volume leads to increase intermolecular interaction and components of mixture are tightly bound to each other.
The decrease in free length with rise in molar concentration of Glycine leads to the compactness and solute -solvent interaction in solution.
The rise in value of η indicates increase in force of attraction between molecules. The force of attraction is dominant than repulsive forces. In present investigation, it is observed that the solute –solvent molecules interacting strongly and forming compact structure. This leads to development of strong intermolecular forces among constituent molecules of solution. Hence stronger interactions form strong hydrogen bond. Glycine is effective on saline soil in view to sustainable farming therefore it can improve the physical properties of saline soil.
Acknowledgement
I am expressing our deepest gratitude and appreciation for the assistance and support received throughout the completion of this research paper. I would like to thank Dr. U.P. Manik for her invaluable guidance, encouragement, and support throughout this research endeavour.
Conflict of Interest
The author declare that they have no known competing financial interests or personal relationship that could have appeared to influence the work reported in this paper
Funding Sources
The author(s) received no financial support for the research, authorship, and/or publication of this article.
Referrences
- Majumder S., Sinha A., Roy D., Ghosh B., Ghosh N.N., Ray T., Dakua V.K., Datta A., Sarkar I.B., Choudhury S., Roy A., Nitish Roy N., Roy M.N.*, ACS Omega, 2023, vol-8, 13, pp 12098–12123, https://doi.org/10.1021/acsomega.2c08008.
CrossRef - Praharaj M.K. , NSA, 2019, pp 1- 4,.https://doi.org/10.13140/RG.2.2. 14684.7 4886.
- Sharma, S., Singh, M., Sharma, S., Singh, J., Sharma,A.K. Sharma, M., Journal of Molecular Liquids,2020, Vol- 303, https://doi.org/10.1016/ j.molliq.2020.112596.
CrossRef - Devi S.,Kumar M., .Sawhney N., Syal U.,Sharma A.K., Sharma M., The Journal of Chemical Thermodynamics, 2021, Vol-154, https://doi.org/10.1016/j.jct.2020.106321.
CrossRef - Arya H.,, Bhatt T., SN Applied Sciences, 2020, Vol-2(1). https://link.springer.com/article/10.1007/s42452-019-1777-5#citeas.
- Nico Eisenhauer N., Bender S.F., Calderón-Sanou I., Franciska T. de Vries, J.J., Thuiller W., Wall D.H., Zeiss R., Bahram M., Beugnon R., Burton V.J., Thomasa W.C., , Delgado-Baquerizo M., Geisen S., Paul K., Kardol, Krashevska V., Carlos A. Martínez-Muñoz, Patoine G., Seeber J., Nadejda A. Soudzilovskaia, Steinwandter M., Sünnemann M., Xin Sun, Marcel G. A. van der HeijdenV.D, GuerraC.A., PotapovA., Journal of sustainable Agriculture and Enviorment,2022, Vol-4,pp 245261, https://doi.org/10.1002/sae2.12031.
CrossRef - Kumar.P., Sharma P.K., Frontiers in Sustainable Food Systems. Frontiers MediaS.A.,2020, Vol-4, https://doi.org/10.3389/fsufs.2020.533781.
CrossRef - Wang T., Liu Q., Wang N., Dai J., Lu Q., Jia X., Lin L., Yu F., Zuo Y., Plant Growth Regulation,2021, https://doi.org/10.1007/s10725-021-00752-2.
CrossRef - Khedr E. , Egypt Journal of Horticulture, 2018 ,Vol-45(1)pp93-103. https://doi.org/10.21608/ejoh.2018.3313.1057.
CrossRef - Tajabadipour A., Reza M., Moghadam F., Zamani Z., Nasibi F., Hokmabadi H., Journal of Nuts ,2018, Vol. 9(1). https://sid.ir/paper/ 716153/en.
- Noroozlo Y.A., Souri M.K., Delshad M.,, Open Agriculture. 2019, Vol-4(1), pp 164–172. https://doi.org/10.1515/opag-2019-0016.
CrossRef - Gupta P., Rai R., Vasudev S., Yadava D.K., Dash P., Journal of Biotechnology,2021, Vol-337, pp 80-89,https://doi.org/10.1016/ j.jbiotec.2021.06.012
CrossRef - Mohammadipour N., Souri M.K., Journal of Plant Nutrition, 2018, vol- 42:14, pp 1637-1644.DOI:10.1080/01904167.2019.1628985
CrossRef - Trovato M., Funk D.,Forlani G., Okumoto S.,Amir R., Frontiers Plant Science, 2021, DOI: 10.3389/fpls.2021.772810.
CrossRef - Patyar P.,Singh G., Kaur K., Journal of molecular Liquids, 2016, Vol-213,pp191-200. https://doi.org/10.1016/j.molliq.2015.11.023
CrossRef - Thirumaran S.*, Karthikeyan N., Oriental Journal of Chemistry ,2014, Vol. 30, No. (1): PP. 133-148. http://dx.doi.org/10.13005/ojc/300116.
CrossRef - Kumari L. , Hussain A. , Agarwal H. , Mishra A. , Gautam4 R., Singh R. , Gautam6 Y., Singh M. , Sinha L. , Onkar Prasad O. Gupta M., Oriental Journal Of Chemistry, 2023, Vol-40(2). http://dx.doi.org//10.13005/ ojc/400209.
CrossRef - Padmanaban. R., Gayathri A., Gopalan A.I., Lee D.-E., Venkatramanan K., Appl. Sci. 2023, Vol-13, 7475. DOI: https://doi.org/10.3390/ app13137475
CrossRef - Singh S., Dhal K., Talukdar M., Biointerface Research. In Applied Chemistry, 2020, Volume 10, (5), PP 6377 – 6388. https://doi.org/ 10.33263/BRIAC105.63776388
CrossRef - Muchipali S., Dayananda S., Priyaranjan M., Nanda B.B., Biointerface Research in Applied Chemistry, 2020, Vol 10(2), PP 5259 – 5265. https://doi.org/10.33263/BRIAC102.259265.
CrossRef - Mistry A. , Bhandakkar V.D. , Chimankar O.P., IOSR Journal of Applied Physics, 2014, PP 1-3. http://www.iosrjournals.org/.
- Khasanshin T.S., Golubeva N.V.,Samuilov V.S., A. P. Shchemelev A.P., Thermophysical Properties of Materials,2017, Vol 55, pP 685–692. DOI: https://link.springer.com/article/10.1134/S0018151X17030117#citeas.
CrossRef - Koirala, B., Dhobi, S. H., Subedi, P., Gurung, M., Teemilsina, N. K., International Journal of Scientific Research in Physics and Applied Sciences,2022, Vol-10(4), pp 8–14.DOI: https://www.researchgate.net/ publication/364820721
This work is licensed under a Creative Commons Attribution 4.0 International License.