Thickening Performance Evaluation and Molecular Dynamics Simulation on Hydrophobic Associated Polymer in Acid Circumstances
Xianwu Jing1,2*, Youquan Liu1
, Lang Zhou3
, Xing Yang3, Pengfei Zhang 1, Jian Lan4, Jie Qi4, Shanshan Dai 4,5,6, Hongsheng Lu4,5,6
1Research Institute of Natural Gas Technology, PetroChina Southwest Oil and Gasfield Company, Chengdu, Sichuan, People’s Republic of China.
2Shale Gas Evalution and Exploitation Key Laboratory of Sichuan Province, Chengdu, Sichuan, People’s Republic of China.
3Engineering Technology Department, PetroChina Southwest Oil and Gasfield Company, Chengdu, Sichuan, People’s Republic of China.
4College of Chemistry and Chemical Engineering, Southwest Petroleum University, Chengdu, People’s Republic of China.
5Oil and Gas Field Applied Chemistry Key Laboratory of Sichuan Province, Chengdu, People’s Republic of China.
6Engineering Research Center of Oilfield Chemistry, Ministry of Education, Chengdu, People’s Republic of China.
Corresponding Author E-mail: jingxw2018@petrochina.com.cn
DOI : http://dx.doi.org/10.13005//ojc/390501
Article Received on : 08 Sep 2023
Article Accepted on : 23 Oct 2023
Article Published : 31 Oct 2023
Reviewed by: Dr. M. Okamoto
Second Review by: Dr. Abdul Aziz Abdul Raman
Final Approval by: Dr. Pounraj Thanasekaran
Acid thickener is of necessity for improving the exploitation capacity for oil and gas resources of carbonate reservoirs. Here a hydrophobic associated polymer (HPA) by introducing a nonionic hydrophobic monomer (AO) was designed as the acid thickener. The experimental results demonstrated that HPA suggests excellent acid resistance, shear resistance, and thermal stability attributing to the gradual exposure of hydrophobic chain to H2O circumstances that enhances the strength of hydrophobic association. The square radius of gyration of HPA raises with the introduction of AO confirming the unfold of HPA by intermolecular hydrophobic association, which strengthens the network structure. The decrease of mean square displacement and diffusion coefficient of H2O and H3O+ molecules suggest the enhancement for network structure by hydrophobic chain owing to HPA hindered the diffusion of H2O and H3O+ molecules. These findings are expected to supply a guidance for the design and research on the acid thickener.
KEYWORDS:Acid thickener; Hydrophobic associated polymer; Intermolecular association; Molecular dynamics simulation
Download this article as:
Copy the following to cite this article: Jing X, Liu Y, Zhou L, Yang Z, Zhang P, Lan Z, Qi J, Dai S, Lu H. Thickening Performance Evaluation and Molecular Dynamics Simulation on Hydrophobic Associated Polymer in Acid Circumstances. Orient J Chem 2023;39(5). |
Copy the following to cite this URL: Jing X, Liu Y, Zhou L, Yang Z, Zhang P, Lan Z, Qi J, Dai S, Lu H. Thickening Performance Evaluation and Molecular Dynamics Simulation on Hydrophobic Associated Polymer in Acid Circumstances. Orient J Chem 2023;39(5). Available from: https://bit.ly/3SoodSq |
Introduction
The ever-increasing exploration on oil and gas reservoir brings abundant fossil fuels and resources. The oil reservoir is mainly consisted of volcanic rock reservoir, clastic rock reservoir and carbonate reservoir. Among these reservoirs, carbonate reservoir is massive and especially rich in oil and gas resources1. Notably, the extraction forcarbonate reservoir poses various challenges due to the particular reservoir conditions, including the extreme burial depth, high temperature, low permeability and porosity2. The reservoir stimulation is admitted to be a desirable strategy to reduce the operation cost and enhance the extraction efficiency3,4. So far, various technologies for carbonate reservoir stimulation have been developed, including drilling stimulation technology, injection of water or gas to maintain formation pressure, and acidizing fracturing technology5. Of which, acidizing fracturing is the most adoptive measurement for stimulating the carbonate reservoir6. This stimulation measurement is realized by etching the carbonatite surface and fracture to improve the flow conductivity and enhance the extraction efficiency for fossil fuels and resources, by using acid solution, such as HCl and HF solution. Traditionally, the acid solution features extremely corrosion property, which brings damages to the reservoirs, environment, and human safety. More importantly, the capacity for carbonate reservoir stimulation is always limited owing to the rapid rate of acid-rock reaction that hinders the extend of cracks, which reduces the depth of acidizing fracturing7. Various acid solution systems have been proposed to slow down the rate of acid-rock reaction by enhancing the viscosity of acid solution for improving the performance on reservoir stimulation, such as, emulsified acid8, foam acid9, diverting acid10, cross-linked acid11, and gelling acid12. Among these acid solution systems, gelling acid is broadly applied on the reservoir stimulation attributing to the excellent thickening capacity, simplicity for preparation and operation.
Gelling acid is a composite solution consisted of acid, polymers, and additives, where polymers, known as acid thickener, play the role on enhancing the viscosity of solution to remit the release rate of acid from solution, which benefits to reducing the filtration loss and extend the acidification scope13. Mostly, acid thickener is consisted of natural high-molecular polymers and synthetic high-molecular polymers. Natural high-molecular polymers, such as guanidine gum14, konjac gum, and carboxymethylcellulose15, poses defects owing to the poor temperature resistance, decomposition by bacteria, and formation of abundant residue. Synthetic high-molecular polymer is a promising choice for evading the existing challenges. High-molecular polymers is always base on the polyacrylamide (PAM) structure, attributing from the better structure stability due to the higher C-C bond energy compared with natural high-molecular polymers16. Previous research has reported a series of synthetic high-molecular polymers for acid thickening17-19. Notably, the exploration for oil resources has turned to ultra-deep carbonate reservoir, which puts forward extra demands on the acid thickener. Traditional synthetic high-molecular polymers feature complete hydrophilicity, which leads to the bad temperature resistance owing to the destruction of hydrogen bond of polymers with raising temperature. Fortunately, hydrophobic associated polymers were proposed to improve the temperature resistance performance afterwards20,21. Different from traditional synthetic high-molecular polymers, hydrophobic associated polymers suggests better thickening performance profiting from the supramolecular interaction of hydrophobic chain22. Besides, hydrophobic associated polymers are expected to show better temperature resistance resulting from the gradual exposure of hydrophobic branched chain to H2O circumstances that enhances the strength of hydrophobic association.
Herein, a hydrophobic associated polymers (HPA) was synthesized, by introducing a nonionic hydrophobic monomer (AO) into Acrylamide/2-acrylamide-2-methylpropyl sulfonic acid/Dimethyl Diallyl Ammonium Chloride (AM/AMPS/DMDAAC) ternary polymer system. The acid resistance, temperature resistance, and shear stability of HPA was investigated by evaluated the changes of viscosity under various experimental conditions for confirming the enhancement for thickening performance by the introduction of AO monomer. Besides, the compatibility of HPA with other additives was evaluated for meeting the practical requirements. The molecular dynamics (MD) of the polymer in acid circumstances were proposed to confirm the thickening mechanism of HPA acid thickener. Furthermore, the square radius of gyration and mean square displacement was analyzed for determining the enhancement for the network of HPA in acid circumstances by the intermolecular interactions from hydrophobic chain.
Material and Methods
Materials
Acrylamide (AM, AR), 2-acrylamide-2-methylpropyl sulfonic acid (AMPS, AR), ethanol, and NaOH were purchased from Kelong Reagents Co. Ltd., Chengdu, China. 2-(2-amino-2-imino-1,1-dimethyl-ethyl) azo-2-methyl-propanamidine dihydrochloride (V50, AR), Dimethyl Diallyl Ammonium Chloride (DMDAAC, AR), acryloyl chloride (AC, AR) and OP-9 were purchased from Macklin Biochemical Co., Ltd, Shanghai, China. Nitrogen (N2, 99.0%) were purchased from by Jinli Gas Co., Ltd., Chengdu, China. Deionized water purified by our laboratory was used in whole experiments.
Synthesis of acid thickener
The Synthesis of terpolymer (PA) and hydrophobic associated polymer (HPA) was accomplish by aqueous solution polymerization. Monomers, including 80mol% AM, 10mol% AMPS, and 10mol% DMDAAC were all fully dissolved into deionized water in a 50mL flask by magnetic stirring. Then, the pH of gained monomer solution was adjusted to 6.5-7.0 by addition NaOH. N2 was subsequently bubbling into the solution in an ice-water bath, and V50, as the initiator, was added after bubbling N2 around 30mins. Finally, the flask was move to water bath to proceed free radical polymerization of PA with the temperature keep 55±1℃ (scheme. 1).
![]() |
Scheme 1: The synthetic details for terpolymer (PA). |
The nonionic hydrophobic monomer AO was synthesized by acylation using AC. AO monomer with 0.2mol% was added into the monomer solution after bubbling N2, HPA was finally gained after free radical polymerization by using V50 at 55±1℃ (scheme. 2). The obtained polymer gel was purified with ethanol, dried, and smashed.
![]() |
Scheme 2: The synthesis method for hydrophobic associated polymer (HPA). |
The determination of intrinsic viscosity and average viscosimetric molecular
The intrinsic viscosity and average viscosimetric molecular of acid thickener was determined by Mark-Houwink relationship using an Ubbelohde viscometer23. The acid thickener was dissolved into a 1M NaCl solution at an initial concentration of 0.01g/mL (C0).
Determination of thickening performance of acid thickener in acid solution
The viscosity changes of acid thickener with a shear rate of 170s-1, at the polymer concentration range from 0.1wt% to 1.0wt% in 20wt% HCl solution, was determined to test the thickening performance. The acid resistance of acid thickener was determined the viscosity of acid thickener at the HCl solution concentration range from 5wt% to 25wt%. The shear resistance of acid thickener was evaluated by operating a shear test lasting 120min with the shear rate of 170s-1, where the viscosity was recorded every 20min. The shear resistance was calculated as follow eq (1).
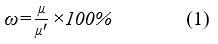
Where, ω (%) stands for the shear stability, μ (mPa·s) stands for the initial viscosity and μ’ (mPa·s) stands for the viscosity of acid thickener sheared after 120min.
Determination of thickening performance of HPA in acid solution
Actually, the compatibility of other additives for acid fracturing is of necessity for the practical application. Here the compatibility of HPA acid thickener with other additives, including 4.0wt% corrosion inhibitor, 1.0wt% iron ion stabilizer, and 1.0wt% cleanup additive were tested. Specifically, the bases of corrosion inhibitor is consisted of Mannich base, iron ion stabilizer iodide and antimony compound, iron ion stabilizer belongs to citric acid, and cleanup additive is consisted of polysorbate surfactant. The compatibility of HPA acid thickener was determined by observing the homogeneous state of the system that consists of acid thickener and the additives in 20wt% HCl solution. The micrographs of the solution were detected to eliminate the adverse effect of color of additives on the experimental accuracy.
Molecular dynamics simulation
The PA and HPA polymer model were built according to the designed structures, where AM, AMPS, DMDAAC, and AO monomers were introduced based on the expected ratio of the synthetic details. The geometry structure of built models was optimized by Forcite module of Materials Studio Soft, and converged to the state that the energy gradient < 0.5 kcal/mol/A and the total energy < 0.001 kcal/mol. The structure of H2O and H3O+ molecules was built at the same way. Furthermore, the cell models of polymers in 20wt% HCl solution were built to confirm the changes of polymers in dynamics. Specifically, the composition of cells was shown in Table. 1. The all-atom MD simulation has been used for the polymer acid solution system, and the system was optimized by MD simulation under NPT ensemble with 200ps in the COMPASS Ⅱ force field of Materials Studio software, an appropriate density of polymer acid solution system was obtained. And then the system was simulated under NVT ensemble with 1200ps, the last 800ps trajectory was taken to investigate the micro behavior of polymer, H2O and H3O+ molecules. The temperature was set to 300K and pressure was 1atm.
Table 1: The composition of cells for terpolymer (PA) and hydrophobic associated polymer (HPA) acid solution.
Composition |
Amount |
Composition |
Amount |
PA |
1 |
HPA |
1 |
H2O |
1616 |
H2O |
1616 |
H3O+ |
394 |
H3O+ |
394 |
Na+ |
10 |
Na+ |
10 |
Cl– |
394+10(0) |
Cl– |
394 |
The parameters, including square radius of gyration (Rg) and mean square displacement (MSD) were utilized investigate the molecular interactions of polymers, H2O, and H3O+. Rg reflects the stretch degree of a polymer chain. Actually, the larger the Rg is, the stronger and the greater the probability for entanglement of polymer molecules, which is more conducive to the formation of dense space network structure and suggests the increase of viscosity at the macro level. The calculation for Rg according to Eq (2).
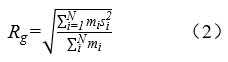
Where, mi stands for the unit mass of polymer and si stands for the distance between atom and the core. N stands for the atom numbers of each polymer.
MSD was defined as the mean values of the square of the displacement and was related to diffusion coefficient (D)24,25. Herein, MSD confirms the diffusion behavior of H2O and H3O+ in acid solution. The smaller the MSD of molecules is, the stronger the constraint of the formed network of polymer on these molecules is. Namely, MSD reflects the strength of the network of polymer. Here both the MSD and D of H2O (and H3O+) were obtained after 800ps MD simulation under NVT ensemble. The relationship between MSD and D was calculated according to Eq (3) and Eq (4).
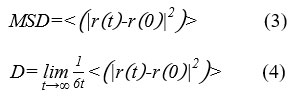
Where, r(t) stands for the coordinates of molecules at time t and r(0) stands for the coordinates of molecules at initial time.
Characterization
The chemical structures of PA and HPA were detected by Fourier Transform Infrared Spectroscopy (FTIR WQF520, Beijing Beifen-Ruili Analytical Instrument Co. Ltd., Beijing, China). The rheological behavior of samples at 160℃ was detected using a rheometer ( HAAKE RS6000, Semer Fisher Technology Co., Ltd, China), where the shear rate keep 170s-1. The viscosity of samples were detected using rotational viscometer (ZNN-D6B, Qingdao Tongchun Petroleum Instrument Co., Ltd, China).
Results and discussion
Characterization of HPA acid thickener
Fig. 1(a) shows the FT-IR spectroscopy of the acid thickener HPA. The peaks appeared at 3467 cm-1 induced by the stretching vibration of N-H and peaks at 1673 cm-1 induced by the stretching vibration of C=O of -CONH2 confirms the existence of AM in the chain segment of HPA. The peaks at 1121 cm-1and 619 cm-1 due to the stretching vibration and deformation vibration of -SO3- verifies that the presence of AMPS. The peaks at 1455 cm-1 introduced by the quaternary ammonium salt indicates the presence of DMDAAC in HPA. Furthermore, the peak appears at 1544 cm-1 caused by the stretching vibration of aromatic ring, and peaks at 873cm-1 attributed to the stretching vibration of P-disubstituted benzene, illustrating the AO monomer was embedded in the HPA chain. The overall results demonstrated the designed acid thickener HPA was synthesized successfully. Similarly, Fig. 1(b) indicates the successfully synthesis of PA. Fig. 1(c) and (d) shows that the lnηr/cr and ηsp/cr were correlated linearly with cr. The intrinsic viscosity of HPA calculated by the y-axis average intercept was determined to be 803.7mL/g, and the average viscosimetric molecular weight of HPA was calculated to be 1.66×106g/mol. Besides, the intrinsic viscosity of PA was determined to be 862.2mL/g, and the average viscosimetric molecular weight of PA was calculated to be 3.74×106g/mol.
![]() |
Figure 1: The FT-IR spectroscopy of hydrophobic associated polymer (HPA) (a) and terpolymer (PA) (b). The Variation of cr of hydrophobic associated polymer (HPA) |
The thickening performance of HPA in acid solution
To meet the demand for the deep acid fracturing construction, the acid thickener is required to exhibit good acid resistance and stability. The the thickening performance of PA and HPA was estimated (see Fig. 2). The viscosity changes of polymer PA and HPA with the concentration range from 0.1 to 1.0wt% was shown in Fig. 2(a). The viscosity of PA varied significantly with the concentration, and reached the maximum value of 51mPa•s at the concentration of 1.0wt%. It is noted that the viscosity of HPA solutions increased sharply with the concentration higher than 0.4wt% and exceeded that of PA. HPA shows excellent hanging feature at the concentration of 0.8wt% and reached the maximum viscosity of 78mPa•s at the concentration of 1.0wt%. Actually, HPA formed weak network structure with the concentration lower than 0.4wt%, which relies on the intramolecular hydrophobic association of hydrophobic side chain, instead of intermolecular hydrophobic association. The hydrophobic branched chain in the HPA can hardly enhance the network structure, attributing to the crimps of HPA polymer molecules caused by the intramolecular hydrophobic association. That is, the hydrophobic branched chain destructed the thickening performance compared with polymer PA. As the HPA concentration increased unceasingly, the network structure gets stronger due to the transformation of the supramolecular interaction form intramolecular to intermolecular hydrophobic association, which results in the sharp increase of viscosity (see Fig. 3) . Furthermore, the acid resistance performance of polymers was evaluated, as shown in Fig. 2(b). The viscosity of HPA decrease by dissolving in HCl solution but keep higher than PA, which attributes to the contribution of intermolecular hydrophobic association from HPA. Notably, the main polymer chain of HPA got crimp with the introduction of HCl resulted in the electrostatic shield effect of H3O+ which destroys the network structure and results in the decrease of viscosity of HPA acid thickener. The viscosity changes slightly with the concentration of HCl solution higher than 15wt%, and the viscosity remained 45mPa•s at the HCl concentration of 25wt%. The polarity of solution was enhanced by increasing HCl concentration, which facilitates the intermolecular hydrophobic association provided by the hydrophobic branched chain attributes to the expand of the polarity difference between side chain and solution. The solubility ability of polymers in 20wt% HCl solution was investigated by evaluating the viscosity changes with time (see Fig. 2(c)). The viscosity of HPA reached the maximum viscosity of 45mPa·s at 60mins, but PA was dissolved completely within 20mins with the maximum viscosity of 36mPa·s. It is admitted that the hydrophobic branched chain in HPA leaded to the decrease of the dissolution performance inevitably, but HPA suggested better thickening performance than PA after being dissolved adequately. Fig. 2(d) shows that the viscosity of HPA and PA in 20wt% HCl solution decreased by only 6.67% and 8.33% after a 120min shear test with a shear rate of 170s-1, indicating the stability under shear force. Notably, the viscosity of HPA in HCl solution attributes to the intermolecular hydrophobic association and winding of polymer chains. The slightly changes of the viscosity of HPA indicates the formed network structure of HPA acid thickener kept stability with directional shear action and suggests the good share resistance of HPA. The compatibility of HPA with other additives, including 4.0wt% corrosion inhibitor, 1.0wt% iron ion stabilizer, and 1.0wt% cleanup additive was investigated, as shown in Fig. 4. The introduction of additives suggested no impact on the thickening performance of HPA, where the solution remained in the homogeneous state within 7 days. Notably, the viscosity of HPA solutions slightly decreased from 45mPa·s to 43.5mPa·s after 7days, which contributes from the inevitable degradation of partial HPA polymer molecule by HCl.
The thermal stability of HPA acid thickener were investigated, as shown in Fig. 5(a). PA features poor temperature resistance, resulting from the crimp of polymer chains caused by the break of hydrogen bond at under high temperature. HPA shows better thermal stability than PA, whose viscosity retention remained 53.3% at 160℃. This is because the destruction of HPA network structure was remitted, resulting from the gradual exposure of hydrophobic branched chain to H2O circumstances that enhances the strength of hydrophobic association. The viscosity changes of polymers in 20wt% HCl solution with a shear rate of 170s-1 at 160℃were evaluated, as shown in Fig. 5(b) and (c). HPA shows better shearing resistance performance than PA resulted from the enhanced network structure, whose viscosity remained 20.68mPa·s by shearing in 120mins.
![]() |
Figure 2: Viscosity changes with different conditions: (a) hydrophobic associated polymer (HPA) concentration, (b) HCl concentration, (c) dissolution time, and (d) shear time. |
![]() |
Figure 3: The viscosity enhancement mechanism attributing to the increase of hydrophobic associated polymer (HPA) concentration. |
![]() |
Figure 4: The compatibility of hydrophobic associated polymer (HPA) with additives. |
![]() |
Figure 5: The viscosity of terpolymer (PA) and hydrophobic associated polymer (HPA) at different temperature (a). The viscosity changes of (b) terpolymer (PA) and (c) hydrophobic associatedpolymer (HPA) |
The thickening mechanism of HPA in acid circumstances
The thickening mechanism of HPA in acid circumstances was examined by using molecular dynamics simulation (MD simulation). The square radius of gyration (Rg) of PA and HPA was calculated, as shown in Fig. 6. The Rg of HPA is bigger than that of PA, indicating that the main polymer chain of HPA was unfolded owing to existence of hydrophobic chain. The collision probability of the unfolded polymer chains gets greater compared with PA. That is, the hydrophobic association strengthened the intermolecular interactions, which is consistent with the experimental study. The global snapshots shows the state of aggregation of PA and HPA with 20wt% HCl solution, as shown in Fig. 7. The network structure of HPA was established by the association of intermolecular hydrophobic chain, as showed in the blue circle. Differently, no interchain association observed in the PA indicates the weaker network structure than HPA. Furthermore, Fig. 8 shows the dynamic formation process of the HPA network structure in acid circumstances. Notably, to reflect the dynamic changes of hydrophobic chain of HPA clearly, the rest of the polymer chain except the hydrophobic chain was hidden. The hydrophobic chain started to associate to unite the branched chains within 400ps, and the formed network structure gets optimized afterwards (see the blue circle in Fig. 8). Obviously, the intermolecular hydrophobic association is of significance to form and reinforce the network structure, resulting in the enhancement of the thickening performance in acid circumstances.
The diffusion behavior of H2O and H3O+ molecules in PA and HPA solution was calculated by using mean square displacement (MSD) and diffusion coefficient (D), for detecting the strength of formed network structure of thickener in acid circumstances, as shown in Fig. 9 and Table. 2. The smaller MSD and D of H2O molecules of HPA that of PA demonstrates the HPA acid thickener hindered the diffusion of H2O molecules, resulting from the enhancement for network structure by hydrophobic chain in HPA. The MSD and D of H3O+ molecules in HPA acid solution are smaller than in PA acid solution resulting from the hinderance of network structure of HPA. Furthermore, the hinderance for H3O+ demonstrated that the HPA acid thickener features excellent acid resistance compared with PA acid thickener, which explains the previous experimental finding.
![]() |
Figure 6: The square radius of gyration (Rg) of terpolymer (PA) and hydrophobic associated polymer (HPA) acid thickener. |
![]() |
Figure 7: The global snapshots of terpolymer (PA) and hydrophobic associated polymer (HPA) in 20wt% HCl solution. |
![]() |
Figure 8: The microscopic dynamics of hydrophobic associated polymer (HPA) molecules at different time in acid circumstances. |
![]() |
Figure 9: The mean square displacement (MSD) of (a) H2O and (b) H3O+ in acid thickener solution. |
Table 2: The diffusion coefficient (D) of H2O and H3O+ in acid thickener solution (m2/s).
Acid thickener solution |
D of H2O |
D of H3O+ |
PA |
3.154×10-9 |
1.215×10-9 |
HPA |
2.815×10-9 |
1.077×10-9 |
Conclusions
Acid thickener retards the diffusion of H3O+ onto rock surface to meet the requirement of in-depth acidification. Herein, a hydrophobic associated polymers (HPA) was synthesized by introducing a nonionic hydrophobic monomer AO, which shows potential application on acid thickening. HPA suggests excellent acid resistance at the concentration of 0.8wt% in 20wt% HCl solution whose viscosity reach 45mPa•s, which attributes to the contribution of intermolecular hydrophobic association. The stable viscosity changes of HPA under steady-state shear indicates the good share resistance. Besides, HPA shows thermal stability whose viscosity retention remained 53.3% at 160℃, attributing to the gradual exposure of hydrophobic chain to H2O circumstances that enhances the strength of hydrophobic association. MD was applied to explain the thickening mechanism of HPA in acid circumstances. The increase of Rg with the introduction of AO monomer demonstrates the unfold of HPA caused by the existence of hydrophobic chain, which strengthened the intermolecular interactions by enhancing the collision probability of HPA polymers. The HPA polymer molecules start to associate within 400ps, and the network structure of HPA was established upon the association of hydrophobic chain, by detecting the dynamic behavior of HPA in acid circumstance. The decrease of MSD and D of H2O and H3O+ molecules with the introduction of AO monomer suggests that HPA hindered the diffusion of H2O and H3O+ molecules, which confirms the enhancement for network structure by hydrophobic chain in HPA. The hinderance for H3O+ demonstrated that the HPA features excellent acid resistance, which is corresponding to the experimental finding. Thus, a hydrophobic associated polymers for acid thickening with good acid and temperature resistant performance was developed, and The MD analysis was proposed to reveal the thickening mechanism of HPA in acid circumstances. Our work is expected to supply a guidance for the design and research on the acid thickener.
Acknowledgment
This work was supported by science and technology project of PetroChina exploration and production company:Technical Improvement and Field Test o Horizontal Shale Gas Wells in Southwest China (No. 2023ZS0603).
Conflict of Interest
The authors declare no competing financial interest.
References
- Li, X.; Qin, R.; Ping, H.; Wei, D.;Liu, X. Journal of Geophysics and Engineering. 2018, 15, 830-840.
CrossRef - Chilingar, G.;Long, W. Energy Sources, Part A: Recovery, Utilization, and Environmental Effects. 2017, 39, 1116-1117.
CrossRef - Wang, X.; Sun, Y.; Li, B.; Zhang, G.; Guo, W.; Li, S.; Jiang, S.; Peng, S.;Chen, H. Energy. 2023, 263, 126120.
CrossRef - Lei, Q.; Weng, D.; Xiong, S.; Liu, H.; Guan, B.; Deng, Q.; Yan, X.; Liang, H.;Ma, Z. Petroleum Exploration and Development. 2021, 48, 1198-1207.
CrossRef - Surjaatmadja, J.B.; McDaniel, B.W.; Case, L.R.; East, L.E.;Pyecroft, J.F. SPE Production & Operations. 2008, 23, 158-169.
CrossRef - Chen, Y.; Wang, H.; Wang, Y.;Ma, G. Journal of Natural Gas Science and Engineering. 2020, 81, 103440.
CrossRef - Jia, C.; Huang, T.; Yao, J.; Xing, H.;Zhang, H. Frontiers in Earth Science. 2021, 9, 698086.
CrossRef - Yousufi, M.M.; Elhaj, M.E.M.; Moniruzzaman, M.; Ayoub, M.A.; Nazri, A.B.M.; Husin, H.b.;Saaid, I.b.M. Journal of Petroleum Exploration and Production Technology. 2018, 9, 1119-1133.
CrossRef - Dai, S.; Gong, Y.; Wang, F.;Hu, P. Geofluids. 2019, 2019, 1-11.
CrossRef - Zhao, L.; Chen, X.; Zou, H.; Liu, P.; Liang, C.; Zhang, N.; Li, N.; Luo, Z.;Du, J. Journal of Petroleum Science and Engineering. 2020, 187, 106734.
CrossRef - Zhao, M.; Li, Y.; Xu, Z.; Wang, K.; Gao, M.; Lv, W.;Dai, C. Colloids and Surfaces A: Physicochemical and Engineering Aspects. 2020, 607, 125471.
CrossRef - Wang, Y.; Fan, Y.; Wang, T.; Ye, J.;Luo, Z. Gels. 2022, 8, 449.
CrossRef - Quan, H.; Li, H.; Huang, Z.; Dai, S.;Jiang, J. Journal of Applied Polymer Science. 2015, 132, 41471.
CrossRef - Jiawei, K.; Yan, J.; Kunpeng, Z.;Pengju, R. Journal of Petroleum Science and Engineering. 2020, 184, 106471.
CrossRef - Freire Soares, A.S.; Furtado de Sousa, A.M.; Calçada, L.A.; Scheid, C.M.;Costa Marques, M.R. Journal of Petroleum Science and Engineering. 2021, 200, 108401.
CrossRef - Zhao, G.; Dai, C.; Wang, S.;Zhao, M. Journal of Applied Polymer Science. 2014, 41637.
- Zhou, L.; Zou, C.; Gu, T.; Li, X.;Shi, Y. Journal of Petroleum Science and Engineering. 2017, 149, 65-74.
CrossRef - Pei, Y.; Zhao, L.; Du, G.; Li, N.; Xu, K.;Yang, H. Petroleum. 2016, 2, 399-407.
CrossRef - Ma, X.;Yang, M. Russian Journal of Applied Chemistry. 2019, 92, 1447-1457.
CrossRef - Mao, J.; Xue, J.; Zhang, H.; Yang, X.; Lin, C.; Wang, Q.; Li, C.;Liao, Z. Colloid and Polymer Science. 2022, 300, 569-582.
CrossRef - Fan, M.; Lai, X.; Li, J.; Wang, T.; Wang, L.; Gao, J.; Wen, X.; Liu, G.;Liu, Y. Polymer Bulletin. 2022, 80, 8725-8743.
CrossRef - Jia, W.; Xian, C.; Jia, B.;Wu, J. Journal of Molecular Liquids. 2023, 370, 121017.
CrossRef - Du, A.; Mao, J.; Zhang, H.; Xu, T.; Yang, X.; Lin, C.; Zhang, Y.;Liu, J. Journal of Molecular Liquids. 2021, 341, 117293.
CrossRef - Tranquillo, R.T.; Lauffenburger, D.A.;Zigmond, S.H. Journal of Cell Biology. 1988, 106, 303-309.
CrossRef - Tzvetkova-Chevolleau, T.; Stephanou, A.; Fuard, D.; Ohayon, J.; Schiavone, P.;Tracqui, P. Biomaterials. 2008, 29, 1541-1551.
CrossRef
Abbreviations
The specific description of full-text abbreviations as shown in Table. 3
Table. 3 The specific description of full-text abbreviations
Abbreviations |
Description of abbreviations |
AO |
A nonionic hydrophobic monomer synthesized by acylation using AC and OP-9 |
PA |
A terpolymer thickener synthesized by AM, AMPS and DMDAAC |
HPA |
A Hydrophobic associated polymer thickener synthesized by AM, AMPS, DMDAAC and AO |
MD |
Molecular Dynamics |
NPT |
Constant-temperature, constant-pressure ensemble |
NVT |
Constant-temperature, constant-volume ensemble |
COMPASS Ⅱ |
Condensed-phase Optimized Molecular Potentials for Atomistic Simulation Studies Ⅱ |
Rg |
Square radius of gyration |
MSD |
Mean square displacement |
D |
Diffusion coefficient |
This work is licensed under a Creative Commons Attribution 4.0 International License.