Kinetics and Mechanisms of Ligand-Exchange of Cu(Methionine)2 by Polyamines
Abdul Moheman1*, Showkat Ahmad Bhawani2
and Khwaja Salahuddin Siddiqi3
1Department of Chemistry, Gandhi Faiz-E-Aam College, Shahjahanpur (Affiliated to M J P Rohilkhand University, Bareilly), Uttar Pradesh, India.
2Faculty of Resource Science and Technology, University Malaysia Sarawak, Kota Samarahan, Sarawak, Malaysia.
3Department of Chemistry, Aligarh Muslim University, Aligarh-202002, UP, India.
Corresponding Author E-mail: amohemanappchem@gmail.com
DOI : http://dx.doi.org/10.13005/ojc/380632
Article Received on : 05 Nov 2022
Article Accepted on :
Article Published : 23 Dec 2022
Reviewed by: Dr. Bal krishan Sharma
Second Review by: Dr. Aditi Sharma
Final Approval by: Dr. S.A. Iqbal
The kinetics of ligand substitution reaction of Cu(methionine)2 complex by polyamines has been followed conductometrically above pH 9.0 at 25 oC. Millimolar solution of the Cu(NO3)2 and methionine in 1:2 molar ratio in water shows increase in conductance with time. When a polyamine (en, dien and trien) is added to the complex, the conductance increases at a rapid rate due to substitution of methionine by polyamine leading to the formation of [Cu(polyamine)2]. It was observed that the exchange of methionine by polyamine proceeds in a first order process. Since the rate of the reaction varies with the size of polyamines they can be placed in the following, en > dien > trien. In acidic pH range the absorption spectrum varies negligibly. However, the concentration of methionine does not have any influence on the absorption spectra.
KEYWORDS:Copper nitrate; Ligand exchange; Methionine; Polyamines
Download this article as:
Copy the following to cite this article: Moheman A, Bhawani S. A, Siddiqi K. S. Kinetics and Mechanisms of Ligand-Exchange of Cu(Methionine)2 by Polyamines. Orient J Chem 2022;38(6). |
Copy the following to cite this URL: Moheman A, Bhawani S. A, Siddiqi K. S. Kinetics and Mechanisms of Ligand-Exchange of Cu(Methionine)2 by Polyamines. Orient J Chem 2022;38(6). Available from: https://bit.ly/3HXAYOx |
Introduction
Amino acids are essential ingredients in biological system. Since we are mimicking such systems by reacting metal ions with amino acids in vitro we have avoided chemicals to represent the biological system. Their involvement in copper transport in blood has been reported1, 2. The amine and carboxylate moieties in amino acids allow them to form compounds with different metal ions1, 3-8. Many amino acids also have a metal-binding site in the side chain, which helps them to form metal complexes with a range of structural motifs. Important metal binding sites include groups like the phenol ring of tyrosine, the thiol group of cysteine, and the thioether moiety of methionine5, 9, 10. Although numerous transition metal ions are involved, Cu(II) has been most extensively studied in living system.
Over the past few decades, equilibrium studies of transition metal complexes in water have received a lot of interest due to the special abilities of water to promote reactions and improve selectivity9-14. According to equilibrium investigations of a few transition metal complexes of sulphur containing amino acids, complexes formed with the sulphur as a thiol are more stable than those formed with the sulphur as a thiol ether15, 16.It has been reported that some metal complexes of methionine in which the sulphur is a thiol ether exhibit stability constants and absorption spectra that are extremely comparable to those of glycine, serine, and valine15, 17. Because of this, methionine has been classified as a bidentate ligand with donor groups for nitrogen and carboxylate.
Methionine exists in two ionic forms, 1 and 2 depending on the pH of the medium (Fig. 1). It has been observed that in acidic pH range the absorption spectrum varies negligibly perhaps because of the ionisation form (Fig. 2). It has been reported by Gregorova and co-workers18 that the absorption peak of methionine at 211 nm at pH 2.0 shifts to 217 nm above pH 9.0. However, we have run the electronic spectra of 0.2 mmolar aqueous solution of methionine at pH 2.0 and above pH 9.0 (Fig. 2) and observed that the absorption at 211 does not shift to higher wavelength although the intensity of absorption varies with pH. It is, therefore, suggested that at pH 9.0 and above the mode of ionisation of methionine occurs as shown in Figure 1.
![]() |
Figure 1: Ionization of methionine in (1) acidic and (2) basic media. |
In basic medium above pH 9.0 the carboxylic groups and the nitrogen atom coordinate with the metal ions. Different modes of coordination of methionine have been suggested.
Hawkins and Perrin19 claim that metal-thiol ether bonding also exists in the copper(II) complex of methionine. However, Lenz and Martell16 demonstrate that the thiol ether is not coordinated to any transition metals, including copper, by using comparable stability reasons (II).
This prompted us to study the kinetics of substitution of the methionine in Cu(methionine)2, by ethylenediamine, diethylenetriamine or triethylenetetramine at ambient temperature above pH 9.0. The primary reaction examined in this work is:

It has been shown in the above reaction that the substitution of methionine by polyamine is essentially complete.
![]() |
Figure 2: Electronic spectra of Cu(methionine)2 complexes at different pH values. |
Experimental
Material and Methods
A UV-2100 spectrophotometer (Shimadzu) with a flow cell was used to measure the electronic spectra. Over the wavelength range of 190-350 nm, the absorbance values were taken at intervals of 1 nm. A CM-82T Elico conductivity metre was used for the kinetic studies. Copper nitrate, methionine, ethylenediamine, diethylennetriamine, triethylenetetramine, potassium chloride, boric acid, orthophosphoric acid and sodium hydroxide were of analar grade. The experiment was conducted using double distilled water (DDW).
Reaction Solutions
Millimolar solutionofCu(methionine)2 was freshly prepared under nitrogen. Methionine was added to metal nitrate in order to give 1:2 complexes. Borate buffer was used to keep the pH constant, while KCl was used to keep the ionic strength constant at 0.3 M. In the case of Cu(methionine)2 substitution of methionine by en only was studied. The solutions were deaerated by bubbling nitrogen for 20 min and their conductance was determined in respect to time at 25.0 ± 1.0ºC. The specific rate constant for ligand substitution, Ks, was determined from the slope of the linear fragment of the molar conductance versus time curve. Three distinct molar ratios of the complex and the en, dien, or trien solutions were combined independently. For a total of 1.5 hours, the conductance of each set was measured instantly and every 5 minutes thereafter. After 24 hours, the limiting conductance (Δ∞) was measured.
Results and Discussion
In a 1:2 molar ratio of Cu(NO3)2:methionine and Cu(methionine)2:en, the molar conductance of the solution increases with time (Fig. 3). The specific rate constants for substitution were determined from the linear section of the conductance vs time plot of complexes and found to be 3.9 × 10-3/S-1 and 5.7 × 10-3/S-1 respectively. On plotting log [Δ∞/(Δ∞ ─ ΔM)] against time nearly a straight line was achieved for varied molar ratios of reactants. Two mutually intersecting linear parts of the graph were found in the exchange reaction between methionine and polyamine (Fig. 4). The specific rate constants, K1and K2, for the stepwise replacement of the methionine from the substrate were determined from the slopes of the first and second linear portions of the graphs, respectively, using the method of two mutually intersecting lines. From following equation:
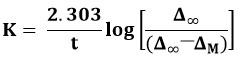
K1 and K2 for the first order rate constants were determined (Table 1). It is clear that en complex with Cu2+ showed high rate constants in comparison to those of diethylenetriamine or triethylenetetramine. The K values decrease with increasing size of the polyamines (Table 1). Perhaps the replacement of a smaller ligand by a larger one is not favored and therefore K values decrease with size of the polyamines. As a result, the amines can be ordered in decreasing order of their rate constants: en > dien > trien.
In the presence of polyamine ligands, the initial rate of rise in molar conductance of the complex may be due to substitution of methionine by polyamine. The exchange of methionineby polyamine may follow either the first order displacement of methionine then a fast addition of polyamine or a second order reaction involving the simultaneous addition of polyamine and cleavage of methionine. But it was found that the reaction in this instance follows the first order pathway (Scheme 1). The polyamine is a stronger ligand than methionine, as evidenced by the rate at which conductance increases throughout the substitution processes. In this experiment, equilibrium was reached after roughly one and half hour.
![]() |
Figure 3: Molar conductance versus time curve for (i) Cu(NO3)2 + 2 methionine → Cu(methionine)2 (ii) Cu(methionine)2 + 2 en → Cu(en)2 in aqueous solution at pH 9.2. |
![]() |
Figure 4: First order plots for the replacement of methionine in [Cu(methionine)2] by ethylenediamine in different molar ratios. |
![]() |
Scheme 1 Click here to View scheme |
Table 1: Rate constant for methionine replacement by polyamines in the Cu(methionine)2 complex.
Complex |
Polyamines |
Ratio |
K1 × 10-3 (S-1) |
K2 × 10-3 (S-1) |
Cu(methionine)2 |
||||
en |
1 : 1 |
1.4 |
5.7 |
|
1 : 2 |
1.4 |
5.7 |
||
1 : large |
1.4 |
5.7 |
||
dien |
1 : 1 |
1.3 |
5.4 |
|
1 : 2 |
1.3 |
5.4 |
||
1 : large |
1.3 |
5.4 |
||
trien |
1 : 1 |
1.2 |
5.2 |
|
1 : 2 |
1.2 |
5.2 |
||
1 : large |
1.2 |
5.2 |
Conclusions
The replacement of methionine by polyamines (ethylenediamine, diethylenetriamine or triethylenetetramine) in copper(methionine)2 is more rapid and has the kinetic properties of an octahedral copper complex. It has been observed that absorption spectrum of methionine varies negligibly in acidic pH range. However, the concentration of methionine does not have any influence on the absorption spectra. Furthermore, our reaction conditions only required water as the solvent which is more environment friendly.
Acknowledgement
No specific grant for this research was provided by funding organizations.
Conflict of Interest
There are no conflict of interest.
References
- Laurie S. H. “Comprehensive coordination chemistry”, ed. G. Wilkinson G., R.D. Gillard R. D.; McCleverty J. A., Vol. 2, Pergamon Press, Oxford, 1987, pp 739-776.
- Festa R. A.; Thiele D. J., Current Biology, 2011, 21, R877-83.
CrossRef - McAuliffe C. A.; Quagliano J. V.; Vallarino L. M., Inorganic Chemistry., 1966, 5, 1996-2003.
CrossRef - Kiss T.,“Biocoordination Chemistry: Coordination equilibria in biologically active systems” ed. K. Burger, Ellis Horwood, Chichester, pp 56–134, 1990.
- Laurie S. H., “Handbook of metal–ligand interactions in biological fluids: bioinorganic chemistry”, ed. G. Berthon, Vol. 1, Marcel Dekker, New York, pp 603–619, 1995.
- Shimazaki Y.; Takani M.; Yamauchi O., Dalton Transactions., 2009, 41, 7854-7869.
CrossRef - Aizawa S.; Takizawa K.; Aitani M., RSC Advances., 2019, 9, 25177-25183.
CrossRef - Abendrot M.; Checinska L.; Kusz J.; Lisowska K.; Zawadzka K.; Felczak A.; Kalinowska-Lis U., Molecules., 2020, 25, 951.
CrossRef - Kurzak B.; Matczak-Jon E.; Hoffmann M., Journal of Coordination Chemistry., 1998, 43, 243-255.
CrossRef - Kurzak B.; Kamecka A.; Bogusz K.; Jezierska J.; Wozna A., Polyhedron., 2009, 28, 2403-2410.
CrossRef - Farkas F.; Enyedy E. A.; Micera G.; Garribba E., Polyhedron., 2000, 19,1727-1736.
CrossRef - Kurzak B.; Kamecka A.; Bogusz K.; Jezierska J., Polyhedron, 2007, 26, 4345-4353.
CrossRef - Kamecka A.; Kurzak B.; Jezierska J.; Wozna A., Journal of Solution Chemistry, 40, 2011, 1041-1054.
CrossRef - Cortes-Clerget M.; Yu J.; Kincaid J. R. A.; Walde P.; Gollou F.; Lipshutz B. H., Chemical Science., 2021, 12, 4237.
CrossRef - Libby R. A.; Margerum D. W., Biochemistry, 1965, 4, 619-625.
CrossRef - Lenz G.; Martell A., Biochemistry., 1964, 3, 745-750.
CrossRef - Pelletier S, Journal de Chimie Physique., 1960, 57, 301-305.
CrossRef - Grigorova E.; Bondarev V. L. P.; Kornienko T. S., Russian Journal of General Chemistry., 2010, 80, 2450-2454.
CrossRef - Hawkins C. J.; Perrin D. D., Inorganic Chemistry., 1963,2, 843-849.
CrossRef
This work is licensed under a Creative Commons Attribution 4.0 International License.